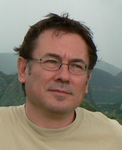
ARROYO Juan
- Dept. Of Plant Biol Ecol., Univ. de Sevilla, Sevilla, Spain
- Adaptation, Evolutionary Ecology, Macroevolution, Phylogenetics / Phylogenomics, Phylogeography & Biogeography, Population Genetics / Genomics, Reproduction and Sex, Speciation, Species interactions
- recommender
Recommendations: 2
Review: 1
Recommendations: 2
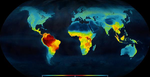
Ancient tropical extinctions contributed to the latitudinal diversity gradient
One (more) step towards a dynamic view of the Latitudinal Diversity Gradient
Recommended by Joaquín Hortal and Juan Arroyo based on reviews by Juan Arroyo, Joaquín Hortal, Arne Mooers, Joaquin Calatayud and 2 anonymous reviewersThe Latitudinal Diversity Gradient (LDG) has fascinated natural historians, ecologists and evolutionary biologists ever since [1] described it about 200 years ago [2]. Despite such interest, agreement on the origin and nature of this gradient has been elusive. Several tens of hypotheses and models have been put forward as explanations for the LDG [2-3], that can be grouped in ecological, evolutionary and historical explanations [4] (see also [5]). These explanations can be reduced to no less than 26 hypotheses, which account for variations in ecological limits for the establishment of progressively larger assemblages, diversification rates, and time for species accumulation [5]. Besides that, although in general the tropics hold more species, different taxa show different shapes and rates of spatial variation [6], and a considerable number of groups show reverse patterns, with richer assemblages in cold temperate regions (see e.g. [7-9]).
Understanding such complexity needs integrating ecological and evolutionary research into the wide temporal and spatial perspectives provided by the burgeoning field of biogeography. This integrative discipline ¬–that traces back to Humboldt himself (e.g. [10])– seeks to put together historical and functional explanations to explain the complex dynamics of Earth’s biodiversity. Different to quantum physicists, biogeographers cannot pursue the ultimate principle behind the patterns we observe in nature due to the interplay of causes and effects, which in fact tell us that there is not such a single principle. Rather, they need to identify an array of basic principles coming from different perspectives, to then integrate them into models that provide realistic –but never simple– explanations to biodiversity gradients such as LDG (see, e.g., [5; 11]). That is, rather than searching for a sole explanation, research on the LDG must aim to identify as many signals hidden in the pattern as possible, and provide hypotheses or models that account for these signals. To later integrate them and, whenever possible, to validate them with empirical data on the organisms’ distribution, ecology and traits, phylogenies, fossils, etc.
Within this context, Meseguer & Condamine [12] provide a novel perspective to LDG research using phylogenetic and fossil evidence on the origin and extinction of taxa within the turtle, crocodile and lizard (i.e. squamate) lineages. By digging into deep time down to the Triassic (about 250 Myr ago) they are able to identify several episodes of flattening and steepening of the LDG for these three clades. Strikingly, their results show similar diversification rates in the northern hemisphere and in the equator during the over 100 Myr long global greenhouse period that extends from the late Jurassic to the Cretaceous and early Neogene. During this period, the LDG for these three groups would have appeared quite even across a mainly tropical Globe, although the equatorial regions were apparently much more evolutionarily dynamic. The equator shows much higher rates of origination and extinction of branches throughout the Cretaceous, but they counteract each other so net diversification is similar to that of the northern hemisphere in all three groups. The transition to a progressively colder Earth in the Paleogene (starting around 50 Myr ago) provokes a mass extinction in the three clades, which is compensated in the equator by the dispersal of many taxa from the areas that currently pertain to the Holarctic biogeographical realm. Finally, during the coldhouse Earth’s climatic conditions of the Neogene only squamates show significant positive diversification rates in extratropical areas, while the diversity of testudines remains, and crocodiles continue declining progressively towards oblivion in the whole world.
Meseguer & Condamine [12] attribute these temporal patterns to the so-called asymmetric gradient of extinction and dispersal (AGED) framework. Here, the dynamics of extinction-at and dispersal-from high latitudes during colder periods increase the steepness of the LDG. Whereas the gradient flattens when Earth warms up as a result of dispersal from the equator followed by increased diversification in extratropical regions. This idea in itself is not new, for the influence of climatic oscillations on diversification rates is well known, at least for the Pleistocene Ice Ages [13], as is the effect of niche conservatism on the LDG [14]. Nevertheless, Meseguer & Condamine’s AGED provides a synthetic verbal model that could allow integrating the three main types of processes behind the LDG into a single framework. To do this it would be necessary to combine AGED’s cycles of dispersal and diversification with realistic models of: (1) the ecological limits to host rich assemblages in the colder and less productive temperate climatic domains; (2) the variations in diversification rates with shifts in temperature and/or energy regimes; and (3) the geographical patterns of climatic oscillation through time that determine the time for species accumulation in each region.
Integrating these models may allow transposing Meseguer & Condamine’s [12] framework into the more mechanistic macroecological models advocated by Pontarp et al. [5]. This type of mechanistic models has been already used to understand the development of biodiversity gradients through the climatic oscillations of the Pleistocene and the Quaternary (e.g. [11]). So the challenge in this case would be to generate a realistic scenario of geographical dynamics that accounts for plate tectonics and long-term climatic oscillations. This is still a major gap and we would benefit from the integrated work by historical geologists and climatologists here. For instance, there is little doubt about the progressive cooling through the Cenozoic based in isotope recording in sea floor sediments [15]. Meseguer & Condamine [12] use this evidence for separating greenhouse, transition and coldhouse world scenarios, which should not be a problem for these rough classes. However, a detailed study of the evolutionary correlation of true climate variables across the tree of life is still pending, as temperature is inferred only for sea water in an ice-free ocean, say earlier half of the Cenozoic [15]. Precipitation regime is even less known. Such scenario would provide a scaffold upon which the temporal dynamics of several aspects of the generation and loss of biodiversity can be modelled. Additionally, one of the great advantages of selecting key clades to study the LDG would be to determine the functional basis of diversification. There are species traits that are well known to affect speciation and extinction probabilities, such as reproductive strategies or life histories (e.g. [16]). Whereas these traits might also be a somewhat redundant effect of climatic causes, they might foster (i.e. “extended reinforcement”, [17]) or slow diversification. Even so, it is unlikely that such a model would account for all the latitudinal variation in species richness. But it will at least provide a baseline for the main latitudinal variations in the diversity of the regional communities (sensu [18]) worldwide. Within this context the effects of recent ecological, evolutionary and historical processes, such as environmental heterogeneity, current diversification rates or glacial cycles, will only modify the general LDG pattern resulting from the main processes contained in Meseguer & Condamine’s AGED, thereby providing a more comprehensive understanding of the geographical gradients of diversity.
References
[1] Humboldt, A. v. (1808). Ansichten der Natur, mit wissenschaftlichen Erläuterungen. J. G. Cotta, Tübingen.
[2] Hawkins, B. A. (2001). Ecology's oldest pattern? Trends in Ecology & Evolution, 16, 470. doi: 10.1016/S0169-5347(01)02197-8
[3] Lomolino, M. V., Riddle, B. R. & Whittaker, R. J. (2017). Biogeography. Fifth Edition. Sinauer Associates, Inc., Sunderland, Massachussets.
[4] Mittelbach, G. G., Schemske, D. W., Cornell, H. V., Allen, A. P., Brown, J. M., Bush, M. B., Harrison, S. P., Hurlbert, A. H., Knowlton, N., Lessios, H. A., McCain, C. M., McCune, A. R., McDade, L. A., McPeek, M. A., Near, T. J., Price, T. D., Ricklefs, R. E., Roy, K., Sax, D. F., Schluter, D., Sobel, J. M. & Turelli, M. (2007). Evolution and the latitudinal diversity gradient: speciation, extinction and biogeography. Ecology Letters, 10, 315-331. doi: 10.1111/j.1461-0248.2007.01020.x
[5] Pontarp, M., Bunnefeld L., Cabral, J. S., Etienne, R. S., Fritz, S. A., Gillespie, R. Graham, C. H., Hagen, O., Hartig, F., Huang, S., Jansson, R., Maliet, O., Münkemüller, T., Pellissier, L., Rangel, T. F., Storch, D., Wiegand, T. & Hurlbert, A. H. (2019). The latitudinal diversity gradient: novel understanding through mechanistic eco-evolutionary models. Trends in ecology & evolution, 34, 211-223. doi: 10.1016/j.tree.2018.11.009
[6] Hillebrand, H. (2004). On the generality of the latitudinal diversity gradient. The American Naturalist, 163, 192-211. doi: 10.1086/381004
[7] Santos, A. M. C. & Quicke, D. L. J. (2011). Large-scale diversity patterns of parasitoid insects. Entomological Science, 14, 371-382. doi: 10.1111/j.1479-8298.2011.00481.x
[8] Morinière, J., Van Dam, M. H., Hawlitschek, O., Bergsten, J., Michat, M. C., Hendrich, L., Ribera, I., Toussaint, E. F. A. & Balke, M. (2016). Phylogenetic niche conservatism explains an inverse latitudinal diversity gradient in freshwater arthropods. Scientific Reports, 6, 26340. doi: 10.1038/srep26340
[9] Weiser, M. D., Swenson, N. G., Enquist, B. J., Michaletz, S. T., Waide, R. B., Zhou, J. & Kaspari, M. (2018). Taxonomic decomposition of the latitudinal gradient in species diversity of North American floras. Journal of Biogeography, 45, 418-428. doi: 10.1111/jbi.13131
[10] Humboldt, A. v. (1805). Essai sur la geographie des plantes; accompagné d'un tableau physique des régions equinoxiales. Levrault, Paris.
[11] Rangel, T. F., Edwards, N. R., Holden, P. B., Diniz-Filho, J. A. F., Gosling, W. D., Coelho, M. T. P., Cassemiro, F. A. S., Rahbek, C. & Colwell, R. K. (2018). Modeling the ecology and evolution of biodiversity: Biogeographical cradles, museums, and graves. Science, 361, eaar5452. doi: 10.1126/science.aar5452
[12] Meseguer, A. S. & Condamine, F. L. (2019). Ancient tropical extinctions contributed to the latitudinal diversity gradient. bioRxiv, 236646, ver. 4 peer-reviewed and recommended by PCI Evol Biol. doi: 10.1101/236646
[13] Jansson, R., & Dynesius, M. (2002). The fate of clades in a world of recurrent climatic change: Milankovitch oscillations and evolution. Annual review of ecology and systematics, 33(1), 741-777. doi: 10.1146/annurev.ecolsys.33.010802.150520
[14] Wiens, J. J., & Donoghue, M. J. (2004). Historical biogeography, ecology and species richness. Trends in ecology & evolution, 19, 639-644. doi: 10.1016/j.tree.2004.09.011
[15] Zachos, J. C., Dickens, G. R., & Zeebe, R. E. (2008). An early Cenozoic perspective on greenhouse warming and carbon-cycle dynamics. Nature, 451, 279-283. doi: 10.1038/nature06588
[16] Zúñiga-Vega, J. J., Fuentes-G, J. A., Ossip-Drahos, A. G., & Martins, E. P. (2016). Repeated evolution of viviparity in phrynosomatid lizards constrained interspecific diversification in some life-history traits. Biology letters, 12, 20160653. doi: 10.1098/rsbl.2016.0653
[17] Butlin, R. K., & Smadja, C. M. (2018). Coupling, reinforcement, and speciation. The American Naturalist, 191, 155-172. doi: 10.1086/695136
[18] Ricklefs, R. E. (2015). Intrinsic dynamics of the regional community. Ecology letters, 18, 497-503. doi: 10.1111/ele.12431
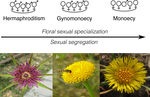
Architectural traits constrain the evolution of unisexual flowers and sexual segregation within inflorescences: an interspecific approach
Sometimes, sex is in the head
Recommended by Juan Arroyo based on reviews by 3 anonymous reviewersPlants display an amazing diversity of reproductive strategies with and without sex. This diversity is particularly remarkable in flowering plants, as highlighted by Charles Darwin, who wrote several botanical books scrutinizing plant reproduction. One particularly influential work concerned floral variation [1]. Darwin recognized that flowers may present different forms within a single population, with or without sex specialization. The number of species concerned is small, but they display recurrent patterns, which made it possible for Darwin to invoke natural and sexual selection to explain them. Most of early evolutionary theory on the evolution of reproductive strategies was developed in the first half of the 20th century and was based on animals. However, the pioneering work by David Lloyd from the 1970s onwards excited interest in the diversity of plant sexual strategies as models for testing adaptive hypotheses and predicting reproductive outcomes [2]. The sex specialization of individual flowers and plants has since become one of the favorite topics of evolutionary biologists. However, attention has focused mostly on cases related to sex differentiation (dioecy and associated conditions [3]). Separate unisexual flower types on the same plant (monoecy and related cases, rendering the plant functionally hermaphroditic) have been much less studied, apart from their possible role in the evolution of dioecy [4] or their association with particular modes of pollination [5].
Two specific non-mutually exclusive hypotheses on the evolution of separate sexes in flowers (dicliny) have been proposed, both anchored in Lloyd’s views and Darwin’s legacy, with selfing avoidance and optimal limited resource allocation. Intermediate sex separation, in which sex morphs have different combinations of unisexual and hermaphrodite flowers, has been crucial for testing these hypotheses through comparative analyses of optimal conditions in suggested transitions. Again, cases in which floral unisexuality does not lead to sex separation have been studied much less than dioecious plants, at both the microevolutionary and macroevolutionary levels. It is surprising that the increasing availability of plant phylogenies and powerful methods for testing evolutionary transitions and correlations have not led to more studies, even though the frequency of monoecy is probably highest among diclinous species (those with unisexual flowers in any distribution among plants within a population [6]).
The study by Torices et al. [7] aims to fill this gap, offering a different perspective to that provided by Diggle & Miller [8] on the evolution of monoecious conditions. The authors use heads of a number of species of the sunflower family (Asteraceae) to test specifically the effect of resource limitation on the expression of sexual morphs within the head. They make use of the very particular and constant architecture of inflorescences in these species (the flower head or “capitulum”) and the diversity of sexual conditions (hermaphrodite, gynomonoecious, monoecious) and their spatial pattern within the flower head in this plant family to develop an elegant means of testing this hypothesis. Their results are consistent with their expectations on the effect of resource limitation on the head, as determined by patterns of fruit size within the head, assuming that female fecundity is more strongly limited by resource availability than male function.
The authors took on a huge challenge in choosing to study the largest plant family (about 25 thousand species). Their sample was limited to only about a hundred species, but species selection was very careful, to ensure that the range of sex conditions and the available phylogenetic information were adequately represented. The analytical methods are robust and cast no doubt on the reported results. However, I can’t help but wonder what would happen if the antiselfing hypothesis was tested simultaneously. This would require self-incompatibility (SI) data for the species sample, as the presence of SI is usually invoked as a powerful antiselfing mechanism, rendering the unisexuality of flowers unnecessary. However, SI is variable and frequently lost in the sunflower family [9]. I also wonder to what extent the very specific architecture of flower heads imposes an idiosyncratic resource distribution that may have fixed these sexual systems in species and lineages of the family. Although not approached in this study, intraspecific variation seems to be low. It would be very interesting to use similar approaches in other plant groups in which inflorescence architecture is lax and resource distribution may differ. A whole-plant approach might be required, rather than investigations of single inflorescences as in this study. This study has no flaws, but instead paves the way for further testing of a long-standing dual hypothesis, probably with different outcomes in different ecological and evolutionary settings. In the end, sex is not only in the head.
References
[1] Darwin, C. (1877). The different forms of flowers on plants of the same species. John Murray.
[2] Barrett, S. C. H., and Harder, L. D. (2006). David G. Lloyd and the evolution of floral biology: from natural history to strategic analysis. In L.D. Harder, L. D., and Barrett, S. C. H. (eds) Ecology and Evolution of Flowers. OUP, Oxford. Pp 1-21.
[3] Geber, M. A., Dawson, T. E., and Delph, L. F. (eds) (1999). Gender and sexual dimorphism in flowering plants. Springer, Berlin.
[4] Charlesworth, D. (1999). Theories of the evolution of dioecy. In Geber, M. A., Dawson T. E. and Delph L. F. (eds) (1999). Gender and sexual dimorphism in flowering plants. Springer, Berlin. Pp. 33-60.
[5] Friedman, J., and Barrett, S. C. (2008). A phylogenetic analysis of the evolution of wind pollination in the angiosperms. International Journal of Plant Sciences, 169(1), 49-58. doi: 10.1086/523365
[6] Renner, S. S. (2014). The relative and absolute frequencies of angiosperm sexual systems: dioecy, monoecy, gynodioecy, and an updated online database. American Journal of botany, 101(10), 1588-1596. doi: 10.3732/ajb.1400196
[7] Torices, R., Afonso, A., Anderberg, A. A., Gómez, J. M., and Méndez, M. (2019). Architectural traits constrain the evolution of unisexual flowers and sexual segregation within inflorescences: an interspecific approach. bioRxiv, 356147, ver. 3 peer-reviewed and recommended by PCI Evol Biol. doi: 10.1101/356147
[8] Diggle, P. K., and Miller, J. S. (2013). Developmental plasticity, genetic assimilation, and the evolutionary diversification of sexual expression in Solanum. American journal of botany, 100(6), 1050-1060. doi: 10.3732/ajb.1200647
[9] Ferrer, M. M., and Good‐Avila, S. V. (2007). Macrophylogenetic analyses of the gain and loss of self‐incompatibility in the Asteraceae. New Phytologist, 173(2), 401-414. doi: 10.1111/j.1469-8137.2006.01905.x
Review: 1
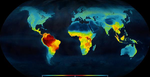
Ancient tropical extinctions contributed to the latitudinal diversity gradient
One (more) step towards a dynamic view of the Latitudinal Diversity Gradient
Recommended by Joaquín Hortal and Juan Arroyo based on reviews by Juan Arroyo, Joaquín Hortal, Arne Mooers, Joaquin Calatayud and 2 anonymous reviewersThe Latitudinal Diversity Gradient (LDG) has fascinated natural historians, ecologists and evolutionary biologists ever since [1] described it about 200 years ago [2]. Despite such interest, agreement on the origin and nature of this gradient has been elusive. Several tens of hypotheses and models have been put forward as explanations for the LDG [2-3], that can be grouped in ecological, evolutionary and historical explanations [4] (see also [5]). These explanations can be reduced to no less than 26 hypotheses, which account for variations in ecological limits for the establishment of progressively larger assemblages, diversification rates, and time for species accumulation [5]. Besides that, although in general the tropics hold more species, different taxa show different shapes and rates of spatial variation [6], and a considerable number of groups show reverse patterns, with richer assemblages in cold temperate regions (see e.g. [7-9]).
Understanding such complexity needs integrating ecological and evolutionary research into the wide temporal and spatial perspectives provided by the burgeoning field of biogeography. This integrative discipline ¬–that traces back to Humboldt himself (e.g. [10])– seeks to put together historical and functional explanations to explain the complex dynamics of Earth’s biodiversity. Different to quantum physicists, biogeographers cannot pursue the ultimate principle behind the patterns we observe in nature due to the interplay of causes and effects, which in fact tell us that there is not such a single principle. Rather, they need to identify an array of basic principles coming from different perspectives, to then integrate them into models that provide realistic –but never simple– explanations to biodiversity gradients such as LDG (see, e.g., [5; 11]). That is, rather than searching for a sole explanation, research on the LDG must aim to identify as many signals hidden in the pattern as possible, and provide hypotheses or models that account for these signals. To later integrate them and, whenever possible, to validate them with empirical data on the organisms’ distribution, ecology and traits, phylogenies, fossils, etc.
Within this context, Meseguer & Condamine [12] provide a novel perspective to LDG research using phylogenetic and fossil evidence on the origin and extinction of taxa within the turtle, crocodile and lizard (i.e. squamate) lineages. By digging into deep time down to the Triassic (about 250 Myr ago) they are able to identify several episodes of flattening and steepening of the LDG for these three clades. Strikingly, their results show similar diversification rates in the northern hemisphere and in the equator during the over 100 Myr long global greenhouse period that extends from the late Jurassic to the Cretaceous and early Neogene. During this period, the LDG for these three groups would have appeared quite even across a mainly tropical Globe, although the equatorial regions were apparently much more evolutionarily dynamic. The equator shows much higher rates of origination and extinction of branches throughout the Cretaceous, but they counteract each other so net diversification is similar to that of the northern hemisphere in all three groups. The transition to a progressively colder Earth in the Paleogene (starting around 50 Myr ago) provokes a mass extinction in the three clades, which is compensated in the equator by the dispersal of many taxa from the areas that currently pertain to the Holarctic biogeographical realm. Finally, during the coldhouse Earth’s climatic conditions of the Neogene only squamates show significant positive diversification rates in extratropical areas, while the diversity of testudines remains, and crocodiles continue declining progressively towards oblivion in the whole world.
Meseguer & Condamine [12] attribute these temporal patterns to the so-called asymmetric gradient of extinction and dispersal (AGED) framework. Here, the dynamics of extinction-at and dispersal-from high latitudes during colder periods increase the steepness of the LDG. Whereas the gradient flattens when Earth warms up as a result of dispersal from the equator followed by increased diversification in extratropical regions. This idea in itself is not new, for the influence of climatic oscillations on diversification rates is well known, at least for the Pleistocene Ice Ages [13], as is the effect of niche conservatism on the LDG [14]. Nevertheless, Meseguer & Condamine’s AGED provides a synthetic verbal model that could allow integrating the three main types of processes behind the LDG into a single framework. To do this it would be necessary to combine AGED’s cycles of dispersal and diversification with realistic models of: (1) the ecological limits to host rich assemblages in the colder and less productive temperate climatic domains; (2) the variations in diversification rates with shifts in temperature and/or energy regimes; and (3) the geographical patterns of climatic oscillation through time that determine the time for species accumulation in each region.
Integrating these models may allow transposing Meseguer & Condamine’s [12] framework into the more mechanistic macroecological models advocated by Pontarp et al. [5]. This type of mechanistic models has been already used to understand the development of biodiversity gradients through the climatic oscillations of the Pleistocene and the Quaternary (e.g. [11]). So the challenge in this case would be to generate a realistic scenario of geographical dynamics that accounts for plate tectonics and long-term climatic oscillations. This is still a major gap and we would benefit from the integrated work by historical geologists and climatologists here. For instance, there is little doubt about the progressive cooling through the Cenozoic based in isotope recording in sea floor sediments [15]. Meseguer & Condamine [12] use this evidence for separating greenhouse, transition and coldhouse world scenarios, which should not be a problem for these rough classes. However, a detailed study of the evolutionary correlation of true climate variables across the tree of life is still pending, as temperature is inferred only for sea water in an ice-free ocean, say earlier half of the Cenozoic [15]. Precipitation regime is even less known. Such scenario would provide a scaffold upon which the temporal dynamics of several aspects of the generation and loss of biodiversity can be modelled. Additionally, one of the great advantages of selecting key clades to study the LDG would be to determine the functional basis of diversification. There are species traits that are well known to affect speciation and extinction probabilities, such as reproductive strategies or life histories (e.g. [16]). Whereas these traits might also be a somewhat redundant effect of climatic causes, they might foster (i.e. “extended reinforcement”, [17]) or slow diversification. Even so, it is unlikely that such a model would account for all the latitudinal variation in species richness. But it will at least provide a baseline for the main latitudinal variations in the diversity of the regional communities (sensu [18]) worldwide. Within this context the effects of recent ecological, evolutionary and historical processes, such as environmental heterogeneity, current diversification rates or glacial cycles, will only modify the general LDG pattern resulting from the main processes contained in Meseguer & Condamine’s AGED, thereby providing a more comprehensive understanding of the geographical gradients of diversity.
References
[1] Humboldt, A. v. (1808). Ansichten der Natur, mit wissenschaftlichen Erläuterungen. J. G. Cotta, Tübingen.
[2] Hawkins, B. A. (2001). Ecology's oldest pattern? Trends in Ecology & Evolution, 16, 470. doi: 10.1016/S0169-5347(01)02197-8
[3] Lomolino, M. V., Riddle, B. R. & Whittaker, R. J. (2017). Biogeography. Fifth Edition. Sinauer Associates, Inc., Sunderland, Massachussets.
[4] Mittelbach, G. G., Schemske, D. W., Cornell, H. V., Allen, A. P., Brown, J. M., Bush, M. B., Harrison, S. P., Hurlbert, A. H., Knowlton, N., Lessios, H. A., McCain, C. M., McCune, A. R., McDade, L. A., McPeek, M. A., Near, T. J., Price, T. D., Ricklefs, R. E., Roy, K., Sax, D. F., Schluter, D., Sobel, J. M. & Turelli, M. (2007). Evolution and the latitudinal diversity gradient: speciation, extinction and biogeography. Ecology Letters, 10, 315-331. doi: 10.1111/j.1461-0248.2007.01020.x
[5] Pontarp, M., Bunnefeld L., Cabral, J. S., Etienne, R. S., Fritz, S. A., Gillespie, R. Graham, C. H., Hagen, O., Hartig, F., Huang, S., Jansson, R., Maliet, O., Münkemüller, T., Pellissier, L., Rangel, T. F., Storch, D., Wiegand, T. & Hurlbert, A. H. (2019). The latitudinal diversity gradient: novel understanding through mechanistic eco-evolutionary models. Trends in ecology & evolution, 34, 211-223. doi: 10.1016/j.tree.2018.11.009
[6] Hillebrand, H. (2004). On the generality of the latitudinal diversity gradient. The American Naturalist, 163, 192-211. doi: 10.1086/381004
[7] Santos, A. M. C. & Quicke, D. L. J. (2011). Large-scale diversity patterns of parasitoid insects. Entomological Science, 14, 371-382. doi: 10.1111/j.1479-8298.2011.00481.x
[8] Morinière, J., Van Dam, M. H., Hawlitschek, O., Bergsten, J., Michat, M. C., Hendrich, L., Ribera, I., Toussaint, E. F. A. & Balke, M. (2016). Phylogenetic niche conservatism explains an inverse latitudinal diversity gradient in freshwater arthropods. Scientific Reports, 6, 26340. doi: 10.1038/srep26340
[9] Weiser, M. D., Swenson, N. G., Enquist, B. J., Michaletz, S. T., Waide, R. B., Zhou, J. & Kaspari, M. (2018). Taxonomic decomposition of the latitudinal gradient in species diversity of North American floras. Journal of Biogeography, 45, 418-428. doi: 10.1111/jbi.13131
[10] Humboldt, A. v. (1805). Essai sur la geographie des plantes; accompagné d'un tableau physique des régions equinoxiales. Levrault, Paris.
[11] Rangel, T. F., Edwards, N. R., Holden, P. B., Diniz-Filho, J. A. F., Gosling, W. D., Coelho, M. T. P., Cassemiro, F. A. S., Rahbek, C. & Colwell, R. K. (2018). Modeling the ecology and evolution of biodiversity: Biogeographical cradles, museums, and graves. Science, 361, eaar5452. doi: 10.1126/science.aar5452
[12] Meseguer, A. S. & Condamine, F. L. (2019). Ancient tropical extinctions contributed to the latitudinal diversity gradient. bioRxiv, 236646, ver. 4 peer-reviewed and recommended by PCI Evol Biol. doi: 10.1101/236646
[13] Jansson, R., & Dynesius, M. (2002). The fate of clades in a world of recurrent climatic change: Milankovitch oscillations and evolution. Annual review of ecology and systematics, 33(1), 741-777. doi: 10.1146/annurev.ecolsys.33.010802.150520
[14] Wiens, J. J., & Donoghue, M. J. (2004). Historical biogeography, ecology and species richness. Trends in ecology & evolution, 19, 639-644. doi: 10.1016/j.tree.2004.09.011
[15] Zachos, J. C., Dickens, G. R., & Zeebe, R. E. (2008). An early Cenozoic perspective on greenhouse warming and carbon-cycle dynamics. Nature, 451, 279-283. doi: 10.1038/nature06588
[16] Zúñiga-Vega, J. J., Fuentes-G, J. A., Ossip-Drahos, A. G., & Martins, E. P. (2016). Repeated evolution of viviparity in phrynosomatid lizards constrained interspecific diversification in some life-history traits. Biology letters, 12, 20160653. doi: 10.1098/rsbl.2016.0653
[17] Butlin, R. K., & Smadja, C. M. (2018). Coupling, reinforcement, and speciation. The American Naturalist, 191, 155-172. doi: 10.1086/695136
[18] Ricklefs, R. E. (2015). Intrinsic dynamics of the regional community. Ecology letters, 18, 497-503. doi: 10.1111/ele.12431