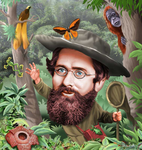
BRISSON Dustin
- Evolution and Ecology of Disease Systems Laboratory, Univ. of Pennsylvania, Philadelphia, United States of America
- Bioinformatics & Computational Biology, Evolutionary Dynamics, Evolutionary Ecology, Evolutionary Epidemiology, Evolutionary Theory, Molecular Evolution, Phylogenetics / Phylogenomics, Phylogeography & Biogeography, Population Genetics / Genomics
- recommender, manager
Recommendation: 1
Reviews: 0
Recommendation: 1
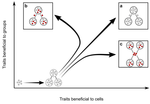
Meta-population structure and the evolutionary transition to multicellularity
The ecology of evolutionary transitions to multicellularity
Recommended by Dustin Brisson based on reviews by 2 anonymous reviewersThe evolutionary transition to multicellular life from free-living, single-celled ancestors has occurred independently in multiple lineages [1-5]. This evolutionary transition to cooperative group living can be difficult to explain given the fitness advantages enjoyed by the non-cooperative, single-celled organisms that still numerically dominate life on earth [1,6,7]. Although several hypotheses have been proposed to explain the transition to multicellularity, a common theme is the abatement of the efficacy of natural selection among the single cells during the free-living stage and the promotion of the efficacy of selection among groups of cells during the cooperative stage, an argument reminiscent of those from George Williams’ seminal book [8,9]. The evolution of life cycles appears to be a key step in the transition to multicellularity as it can align fitness advantages of the single-celled 'reproductive' stage with that of the cooperative 'organismal' stage [9-12]. That is, the evolution of life cycles allows natural selection to operate over timescales longer than that of the doubling time of the free-living cells [13]. Despite the importance of this issue, identifying the range of ecological conditions that reduce the importance of natural selection at the single-celled, free-living stage and increase the importance of selection among groups of cooperating cells has not been addressed empirically.
Rose et al [14] addressed this issue in a series of real time evolution experiments with bacteria in which they varied the intensity of between-group versus individual-level selection. Central to the experiment is an ecological scaffold that requires lineages to switch between free-living (reproductive) and group-living (organismal) life-stages. One ecological scenario severely limited natural selection at the single-celled, free-living stage by maintaining separation among the reproductive propagules originating from different organisms (groups of cells derived from a single ancestral cell). A second ecological scenario mixed the reproductive propagules from different organisms, leading to severe competition between single cells derived from both the same and other 'organisms'. These ecological scenarios lead to very different evolutionary outcomes. Limiting competition, and thus natural selection, at the reproductive propagule stage promoted traits that favored organismal fitness at the expense of cell division, while competition among single-cells favored traits that promote cell-level traits at the expense of group-level traits. The authors investigate a range of measures of cell and group-level performance in order to understand the mechanisms favoring organismal versus single-cell fitness. Importantly, an evolutionary trade-off between traits promoting organismal fitness and single-cell fitness appears to constrain maximizing fitness of both phases, especially when strong natural selection acts on the single-cell stage.
This article is incredibly thorough and utilizes multiple experiments and levels of argument in order to support the conclusions. The authors include considerable discussion of broader topics surrounding the immediate hypotheses throughout the article, which add both clarity and complexity. The complexity of the experiments, results, and the topic itself lead to a thought-heavy article in a throwback to the monographs of old; expect to read each section multiple times.
References
[1] Maynard Smith, J. and Szathmáry, E. (1995). The Major Transitions in Evolution. Oxford, UK: Freeman. p 346.
[2] Bonner, J. T. (1998). The origins of multicellularity. Integrative Biology: Issues, News, and Reviews: Published in Association with The Society for Integrative and Comparative Biology, 1(1), 27-36. doi: 10.1002/(SICI)1520-6602(1998)1:1<27::AID-INBI4>3.0.CO;2-6
[3] Kaiser, D. (2001). Building a multicellular organism. Annual review of genetics, 35(1), 103-123. doi: 10.1146/annurev.genet.35.102401.090145
[4] Medina, M., Collins, A. G., Taylor, J. W., Valentine, J. W., Lipps, J. H., Amaral-Zettler, L., and Sogin, M. L. (2003). Phylogeny of Opisthokonta and the evolution of multicellularity and complexity in Fungi and Metazoa. International Journal of Astrobiology, 2(3), 203-211. doi: 10.1017/S1473550403001551
[5] King, N. (2004). The unicellular ancestry of animal development. Developmental cell, 7(3), 313-325. doi: 10.1016/j.devcel.2004.08.010
[6] Michod R. E. (1999). Darwinian Dynamics. Evolutionary Transitions in Fitness and Individuality. Princeton, NJ: Princeton Univ. Press. p 262.
[7] Lynch, M. (2007). The frailty of adaptive hypotheses for the origins of organismal complexity. Proceedings of the National Academy of Sciences, 104(suppl 1), 8597-8604. doi: 10.1073/pnas.0702207104
[8] Williams, G. C. (1996). Adaptation and Natural Selection, Reprint edition. Princeton, NJ: Princeton Univ. Press.
[9] Grosberg, R. K., and Strathmann, R. R. (2007). The evolution of multicellularity: a minor major transition?. Annu. Rev. Ecol. Evol. Syst., 38, 621-654. doi: 10.1146/annurev.ecolsys.36.102403.114735
[10] Buss, L. W. (1987). The Evolution of Individuality. Princeton, NJ: Princeton Univ. Press.
[11] Godfrey-Smith, P. (2009). Darwinian Populations and Natural Selection. Oxford University Press, USA.
[12] Van Gestel, J., and Tarnita, C. E. (2017). On the origin of biological construction, with a focus on multicellularity. Proceedings of the National Academy of Sciences, 114(42), 11018-11026. doi: 10.1073/pnas.1704631114
[13] Black, A. J., Bourrat, P., and Rainey, P. B. (2020). Ecological scaffolding and the evolution of individuality. Nature Ecology & Evolution, 4(3), 426-436. doi: 10.1038/s41559-019-1086-9
[14] Rose, C. J., Hammerschmidt, K., Pichugin, Y. and Rainey, P. B. (2020). Meta-population structure and the evolutionary transition to multicellularity. bioRxiv, 407163, ver. 5 peer-reviewed and recommended by PCI Evolutionary Biology. doi: 10.1101/407163