BLANCKENHORN Wolf
- Invertebrate Evolutionary Ecology, University of Zurich, Zurich, Switzerland
- Adaptation, Behavior & Social Evolution, Evolutionary Ecology, Experimental Evolution, Life History, Phenotypic Plasticity, Sexual Selection, Speciation
- recommender
Recommendations: 4
Review: 1
Recommendations: 4
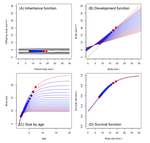
Density dependent environments can select for extremes of body size
A population biological modeling approach for life history and body size evolution
Recommended by Wolf Blanckenhorn based on reviews by Frédéric Guillaume and 2 anonymous reviewersBody size evolution is a central theme in evolutionary biology. Particularly the question of when and how smaller body sizes can evolve continues to interest evolutionary ecologists, because most life history models, and the empirical evidence, document that large body size is favoured by natural and sexual selection in most (even small) organisms and environments at most times. How, then, can such a large range of body size and life history syndromes evolve and coexist in nature?
The paper by Coulson et al. lifts this question to the level of the population, a relatively novel approach using so-called integral projection (simulation) models (IPMs) (as opposed to individual-based or game theoretical models). As is well outlined by (anonymous) Reviewer 1, and following earlier papers spearheading this approach in other life history contexts, the authors use the well-known carrying capacity (K) of population biology as the ultimate fitness parameter to be maximized or optimized (rather than body size per se), to ultimately identify factors and conditions promoting the evolution of extreme body sizes in nature. They vary (individual or population) size-structured growth trajectories to observe age and size at maturity, surivorship and fecundity/fertility schedules upon evaluating K (see their Fig. 1). Importantly, trade-offs are introduced via density-dependence, either for adult reproduction or for juvenile survival, in two (of several conceivable) basic scenarios (see their Table 2). All other relevant standard life history variables (see their Table 1) are assumed density-independent, held constant or zero (as e.g. the heritability of body size).
The authors obtain evidence for disruptive selection on body size in both scenarios, with small size and a fast life history evolving below a threshold size at maturity (at the lowest K) and large size and a slow life history beyond this threshold (see their Fig. 2). Which strategy wins ultimately depends on the fitness benefits of delaying sexual maturity (at larger size and longer lifespan) at the adult stage relative to the preceeding juvenile mortality costs, in agreement with classic life history theory (Roff 1992, Stearns 1992). The modeling approach can be altered and refined to be applied to other key life history parameters and environments. These results can ultimately explain the evolution of smaller body sizes from large body sizes, or vice versa, and their corresponding life history syndromes, depending on the precise environmental circumstances.
All reviewers agreed that the approach taken is technically sound (as far as it could be evaluated), and that the results are interesting and worthy of publication. In a first round of reviews various clarifications of the manuscript were suggested by the reviewers. The new version was substantially changed by the authors in response, to the extent that it now is a quite different but much clearer paper with a clear message palatable for the general reader. The writing is now to the point, the paper's focus becomes clear in the Introduction, Methods & Results are much less technical, the Figures illustrative, and the descriptions and interpretations in the Discussion are easy to follow.
In general any reader may of course question the choice and realism of the scenarios and underlying assumptions chosen by the authors for simplicity and clarity, for instance no heritability of body size and no cost of reproduction (other than mortality). But this is always the case in modeling work, and the authors acknowledge and in fact suggest concrete extensions and expansions of their approach in the Discussion.
References
Coulson T., Felmy A., Potter T., Passoni G., Montgomery R.A., Gaillard J.-M., Hudson P.J., Travis J., Bassar R.D., Tuljapurkar S., Marshall D.J., Clegg S.M. (2022) Density-dependent environments can select for extremes of body size. bioRxiv, 2022.02.17.480952, ver. 3 peer-reviewed and recommended by Peer Community in Evolutionary Biology. https://doi.org/10.1101/2022.02.17.480952
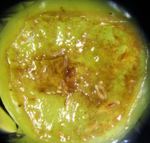
Environmental specificity in Drosophila-bacteria symbiosis affects host developmental plasticity
Nutrition-dependent effects of gut bacteria on growth plasticity in Drosophila melanogaster
Recommended by Wolf Blanckenhorn based on reviews by Pedro Simões and 1 anonymous reviewerIt is well known that the rearing environment has strong effects on life history and fitness traits of organisms. Microbes are part of every environment and as such likely contribute to such environmental effects. Gut bacteria are a special type of microbe that most animals harbor, and as such they are part of most animals’ environment. Such microbial symbionts therefore likely contribute to local adaptation [1]. The main question underlying the laboratory study by Guilhot et al. [2] was: How much do particular gut bacteria affect the organismal phenotype, in terms of life history and larval foraging traits, of the fruit fly Drosophila melanogaster, a common laboratory model species in biology?
To investigate the above question, the authors isolated 4 taxa of bacteria from the gut of a (randomly picked) Drosophila melanogaster lab strain, and subsequently let Drosophila melanogaster eggs and larvae (stemming from their own, different lab strain) develop both in the typical artificial laboratory medium as well as in grapes, a natural “new” habitat for Drosophila larvae, inoculated with theses bacteria, singly and in combination, also including a bacteria-free control. By investigating various relevant developmental and size traits, the authors found that adding particularly Enterobacteria had some visible effects on several traits, both upward (indicting improvement) and downward (being detrimental) (with three other types of bacteria showing only minor or even no effects). In general, the grape medium reduced performance relative to the standard lab medium. Strongest interactive effects occurred for development time and body size, together making up growth plasticity [3], with lesser such effects on some related behavioral (feeding) traits (Figs. 2,3).
The study premise is interesting, its general objectives are clearly laid out, and the practical work was conducted correctly as far as I can evaluate. The study remains largely descriptive in that no particular a priori hypotheses or predictions in relation to the specific bacteria isolated were formulated, not least because the bacteria were necessarily somewhat arbitrarily chosen and there were apparently no prior studies from which to derive concrete predictions. Overall, the results of this study should be of interest to the community of evolutionary ecologists, especially those working on nutritional and microbiome effects on animal life histories. I consider this work to be primarily ecological, with limited evolutionary content (e.g. no genetics) though some evolutionary implications, as mentioned in the paper’s Conclusions. So this paper would best fit in a microbial or physiological ecology outlet/journal.
The inclusion of a natural medium (grapes) must be commended because this permits inferences and conclusions for at least one natural environment, whereas inferences drawn from laboratory studies in the artificial medium that most Drosophila researchers seem to use are typically limited. Unsurprisingly perhaps, the study showed that Drosophila melanogaster fared generally better in the artificial than the chosen natural medium (grape). Crucially, however, the bacterial symbionts modified both media differentially. Although common bacterial taxa were chosen, the particular bacteria isolated and used remain arbitrary, as there are many. I note that the main and strongest interactive effects between medium and bacterial type are apparent for the Enterobacteria, and they probably also strongly, if not exclusively, mediate the overall effect of the bacterial mixture.
While these specific data are novel, they are not very surprising. If we grow animals in different environments we can expect some detectable effects of these environments, including the bacterial (microbiome) environment, on the hosts life history. The standard and predicted [4] life history response of Drosophila melanogaster (but not all insects [3]) facing stressful nutritional environments, as apparently created by the Enterobacteria, is to extend development but come out smaller in the end. This is what happened here for the laboratory medium ([2]: Fig. 5). The biological interpretation is that individuals have more trouble ingesting and/or digesting the nutrients available (thus prolonging their foraging period and development), yet cannot convert the nutrients effectively into body size increments (hence emerging smaller). This is what the authors here refer to as developmental plasticity, which is ultimately nutritionally mediated. However, interestingly, a signal in the opposite direction was indicated for the bacterial mixture in the grape medium (flies emerging larger after accelerated development: Fig. 5), suggesting some positive effects on growth rate of the natural medium, perhaps related to grapes being a limited resource that needs to be escaped quickly [3]? The reversal of sexual size dimorphism across bacterial treatments in the grape environment detectable in Fig. 4 is interesting, too, though I don’t understand why this happens, and this is not discussed.
In general, more encompassing and increased questions in this context to be researched in the future could be: 1) are these effects predictable (not (yet) at this point, or so it seems); and 2) how strong are these environmental bacterial effects relative to other, more standard effects (e.g. relative to genetic variation, population variation, etc., or relative to other types of environmental effects like, say, temperature)? (3) It could further be asked why not natural but laboratory populations of Drosophila were used for this experiment, if the aim was to draw inferences for the wild situation. (4) Although Genotype x Environment effects are invoked in the Discussion, they were not tested here, lacking genetically different Drosophila families or populations. From an evolutionary standpoint, I consider this the greatest weakness of the study. I was also not too thrilled by the particular statistical analyses employed, though this ultimately does not negate the results. Nevertheless, this work is a good start in this huge field investigating the microbiome. In conclusion, I can recommend this paper after review by PCI Evol Biol.
References
[1] Kawecki, T. J. and Ebert, D. (2004) Conceptual issues in local adaptation. Ecology Letters 7: 1225-1241. doi: 10.1111/j.1461-0248.2004.00684.x
[2] Guilhot, R., Rombaut, A., Xuéreb, A., Howell, K. and Fellous, S. (2019). Environmental specificity in Drosophila-bacteria symbiosis affects host developmental plasticity. BioRxiv, 717702, v3 peer-reviewed and recommended by PCI Evolutionary Biology. doi: 10.1101/717702
[3] Blanckenhorn, W.U. (1999) Different growth responses to temperature and resource limitation in three fly species with similar life histories. Evolutionary Ecology 13: 395-409. doi: 10.1023/A:1006741222586
[4] Stearns, S. C. and Koella, J. (1986) The evolution of phenotypic plasticity in life history traits: predictions of reaction norms for age and size at maturity. Evolution 40: 893-914. doi: 10.1111/j.1558-5646.1986.tb00560.x
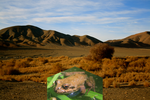
Natural selection on plasticity of thermal traits in a highly seasonal environment
Is thermal plasticity itself shaped by natural selection? An assessment with desert frogs
Recommended by Wolf Blanckenhorn based on reviews by Dries Bonte, Wolf Blanckenhorn and Nadia Aubin-HorthIt is well known that climatic factors – most notably temperature, season length, insolation and humidity – shape the thermal niche of organisms on earth through the action of natural selection. But how is this achieved precisely? Much of thermal tolerance is actually mediated by phenotypic plasticity (as opposed to genetic adaptation). A prominent expectation is that environments with greater (daily and/or annual) thermal variability select for greater plasticity, i.e. better acclimation capacity. Thus, plasticity might be selected per se.
A Chilean group around Leonardo Bacigalupe assessed natural selection in the wild in one marginal (and extreme) population of the four-eyed frog Pleurodema thaul (Anura: Leptodactylidae) in an isolated oasis in the Atacama Desert, permitting estimation of mortality without much potential of confounding it with migration [1]. Several thermal traits were considered: CTmax – the critical maximal temperature; CTmin – the critical minimum temperature; Tpref – preferred temperature; Q10 – thermal sensitivity of metabolism; and body mass. Animals were captured in the wild and subsequently assessed for thermal traits in the laboratory at two acclimation temperatures (10° & 20°C), defining the plasticity in all traits as the difference between the traits at the two acclimation temperatures. Thereafter the animals were released again in their natural habitat and their survival was monitored over the subsequent 1.5 years, covering two breeding seasons, to estimate viability selection in the wild. The authors found and conclude that, aside from larger body size increasing survival (an unsurprising result), plasticity does not seem to be systematically selected directly, while some of the individual traits show weak signs of selection.
Despite limited sample size (ca. 80 frogs) investigated in only one marginal but very seasonal population, this study is interesting because selection on plasticity in physiological thermal traits, as opposed to selection on the thermal traits themselves, is rarely investigated. The study thus also addressed the old but important question of whether plasticity (i.e. CTmax-CTmin) is a trait by itself or an epiphenomenon defined by the actual traits (CTmax and CTmin) [2-5]. Given negative results, the main question could not be ultimately solved here, so more similar studies should be performed.
References
[1] Bacigalupe LD, Gaitan-Espitia, JD, Barria AM, Gonzalez-Mendez A, Ruiz-Aravena M, Trinder M & Sinervo B. 2018. Natural selection on plasticity of thermal traits in a highly seasonal environment. bioRxiv 191825, ver. 5 peer-reviewed by Peer Community In Evolutionary Biology. doi: 10.1101/191825
[2] Scheiner SM. 1993. Genetics and evolution of phenotypic plasticity. Annual Review in Ecology and Systematics 24: 35–68. doi: 10.1146/annurev.es.24.110193.000343
[3] Scheiner SM. 1993. Plasticity as a selectable trait: Reply to Via. The American Naturalist. 142: 371–373. doi: 10.1086/285544
[4] Via S. 1993. Adaptive phenotypic plasticity - Target or by-product of selection in a variable environment? The American Naturalist. 142: 352–365. doi: 10.1086/285542
[5] Via S. 1993. Regulatory genes and reaction norms. The American Naturalist. 142: 374–378. doi: 10.1086/285542
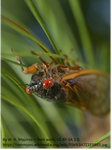
Geographic body size variation in the periodical cicadas Magicicada: implications for life cycle divergence and local adaptation
Megacicadas show a temperature-mediated converse Bergmann cline in body size (larger in the warmer south) but no body size difference between 13- and 17-year species pairs
Recommended by Wolf Blanckenhorn and Thomas FlattPeriodical cicadas are a very prominent insect group in North America that are known for their large size, good looks, and loud sounds. However, they are probably known best to evolutionary ecologists because of their long juvenile periods of 13 or 17 years (prime numbers!), which they spend in the ground. Multiple related species living in the same area are often coordinated in emerging as adults during the same year, thereby presumably swamping any predators specialized on eating them.
Life history differences between the 13yr and 17yr cicadas are a particular focus of interest. For example, as it takes time to grow large, one would expect 17yr cicadas to be larger than 13yr cicadas on average. Koyama et al. [1] investigate geographic body size clines for 7 species of periodical cicadas in eastern North America, whose phylogenetic relationships are resolved, in a life history context, using an impressively large number of populations (Fig. 1 of [1]). The authors report generally female-biased sexual body size dimorphism (SSD), and (however not for all species) a positive relationship of body size with habitat annual mean temperature taken from weather data and a negative correlation with latitude (Fig. 3 of [1]). The latter is consistent with a converse Bergmann cline. Crucially, body size of two at least partly sympatric 13y & 17y sister species pairs did not differ (by much), contrary to expectation because the 17y species have more time to grow larger. 13y cicadas must therefore generally grow faster (or 17y cicadas slower) to in the end acquire the same (optimal?) body size. The phylogenetically oldest 13y cicada species, however, is larger, suggesting that selection for large (optimal?) body size has relaxed over evolutionary time, for unknown reasons (about which the authors speculate). A mechanistic explanation for this phenomenon is suggested based on the hypothesis that 17y cicadas simply arrest or slow down growth early during their juvenile stage to delay emergence for 4 further years (Fig. 2 of [1]).
We think this is an impressive data set, and the life history question addressed in this prominent insect taxon should appeal to readers generally interested in whole-organism evolution despite being largely descriptive.
Reference
[1] Koyama T, Ito H, Kakishima S, Yoshimura J, Cooley JR, Simon C, Sota T. 2015. Geographic body size variation in the periodical cicadas Magicicada: implications for life cycle divergence and local adaptation. Journal of Evolutionary Biology 28:1270-1277. doi: 10.1111/jeb.12653
Review: 1
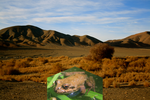
Natural selection on plasticity of thermal traits in a highly seasonal environment
Is thermal plasticity itself shaped by natural selection? An assessment with desert frogs
Recommended by Wolf Blanckenhorn based on reviews by Dries Bonte, Wolf Blanckenhorn and Nadia Aubin-HorthIt is well known that climatic factors – most notably temperature, season length, insolation and humidity – shape the thermal niche of organisms on earth through the action of natural selection. But how is this achieved precisely? Much of thermal tolerance is actually mediated by phenotypic plasticity (as opposed to genetic adaptation). A prominent expectation is that environments with greater (daily and/or annual) thermal variability select for greater plasticity, i.e. better acclimation capacity. Thus, plasticity might be selected per se.
A Chilean group around Leonardo Bacigalupe assessed natural selection in the wild in one marginal (and extreme) population of the four-eyed frog Pleurodema thaul (Anura: Leptodactylidae) in an isolated oasis in the Atacama Desert, permitting estimation of mortality without much potential of confounding it with migration [1]. Several thermal traits were considered: CTmax – the critical maximal temperature; CTmin – the critical minimum temperature; Tpref – preferred temperature; Q10 – thermal sensitivity of metabolism; and body mass. Animals were captured in the wild and subsequently assessed for thermal traits in the laboratory at two acclimation temperatures (10° & 20°C), defining the plasticity in all traits as the difference between the traits at the two acclimation temperatures. Thereafter the animals were released again in their natural habitat and their survival was monitored over the subsequent 1.5 years, covering two breeding seasons, to estimate viability selection in the wild. The authors found and conclude that, aside from larger body size increasing survival (an unsurprising result), plasticity does not seem to be systematically selected directly, while some of the individual traits show weak signs of selection.
Despite limited sample size (ca. 80 frogs) investigated in only one marginal but very seasonal population, this study is interesting because selection on plasticity in physiological thermal traits, as opposed to selection on the thermal traits themselves, is rarely investigated. The study thus also addressed the old but important question of whether plasticity (i.e. CTmax-CTmin) is a trait by itself or an epiphenomenon defined by the actual traits (CTmax and CTmin) [2-5]. Given negative results, the main question could not be ultimately solved here, so more similar studies should be performed.
References
[1] Bacigalupe LD, Gaitan-Espitia, JD, Barria AM, Gonzalez-Mendez A, Ruiz-Aravena M, Trinder M & Sinervo B. 2018. Natural selection on plasticity of thermal traits in a highly seasonal environment. bioRxiv 191825, ver. 5 peer-reviewed by Peer Community In Evolutionary Biology. doi: 10.1101/191825
[2] Scheiner SM. 1993. Genetics and evolution of phenotypic plasticity. Annual Review in Ecology and Systematics 24: 35–68. doi: 10.1146/annurev.es.24.110193.000343
[3] Scheiner SM. 1993. Plasticity as a selectable trait: Reply to Via. The American Naturalist. 142: 371–373. doi: 10.1086/285544
[4] Via S. 1993. Adaptive phenotypic plasticity - Target or by-product of selection in a variable environment? The American Naturalist. 142: 352–365. doi: 10.1086/285542
[5] Via S. 1993. Regulatory genes and reaction norms. The American Naturalist. 142: 374–378. doi: 10.1086/285542