
ALVAREZ Ines
- Department of Biodiversity and Conservation, Royal Botanic Garden, CSIC, Madrid, Spain
- Genotype-Phenotype, Hybridization / Introgression, Phenotypic Plasticity, Phylogenetics / Phylogenomics, Phylogeography & Biogeography, Reproduction and Sex, Speciation, Systematics / Taxonomy
- recommender
Recommendations: 2
Reviews: 0
Recommendations: 2
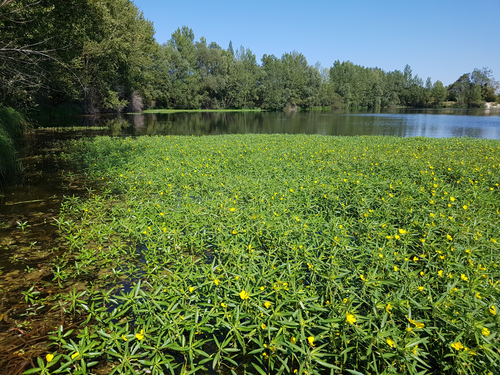
Reproductive modes in populations of late-acting self-incompatible and self-compatible polyploid Ludwigia grandiflora subsp. hexapetala in western Europe
Mixed reproduction modes explain a high genetic diversity in the invasive plant Ludwigia grandiflora subsp. hexapetala in western Europe
Recommended by Ines Alvarez based on reviews by Rubén Torices and 2 anonymous reviewersThe introduction of Ludwigia species as ornamental plants in both North America and Europe dates back almost two centuries, during which time they expanded as naturalized and later invasive species in these territories (Dandelot et al. 2005, Okada et al. 2009). Repeated deliberate or non-deliberate introductions over time of this species complex that can hybridize has given rise to an evolutionarily complex scenario, which is compounded by the difficulty in delimiting some of these species and by the diversity of their modes of reproduction.
Dandelot (2004) and Dandelot et al. (2005) determined the presence of two Ludwigia taxa in France, L. peploides subsp. montevidensis (Spreng.) P.H.Raven (here after Lpm), and L. grandiflora subsp. hexapetala (Hook. & Arn.) G.L.Nesom & Kartesz (here after Lgh), based on their cytotypes (2n = 16, and 2n = 80, respectively) and without evidence of hybridization between them. Furthermore, despite a predominantly vegetative reproduction observed for both species, they differed in their breeding systems. While Lpm is self-compatible and produce a high number of viable seeds in all populations, Lgh is self-incompatible and its populations may drastically differ in seed viability (Dandelot 2004). Several years later, Portillo-Lemus et al. (2021) determined that the differences in seed production between some populations of Lgh are due to the existence of a heteromorphic reproductive system in this taxon, involving a self-incompatible morph (long-style morph; hereafter L-morph), and a self-compatible morph (short-style morph: hereafter S-morph). Moreover, Portillo-Lemus et al. (2022) observed that self-pollen in the L-morph flowers stop growing lately (i.e., in the ovarian area) without fertilizing the ovules, concluding that a late-acting self-incompatible system (hereafter, LSI) is present in this morph.
At this point, it is relevant to understand the possible interactions between populations of different morphs in Lgh, and the implications that they may have on their expansive success in non-native areas in order to develop more effective management plans. To achieve this goal, Stoeckel et al. (2024) analyzed the population genetics in 53 Lgh populations in western Europe (without finding any Lpm population in the sampling area), 40 of which exclusively presented the L-morph and 13 the S-morph. This fact offered the opportunity to compare and interpret the differences between populations of different morphs in Lgh. Other previous works on genetic diversity of Lgh in peripatric or non-native areas pointed to a high clonality and an extremely low genetic diversity (Okada et al. 2009, Armitage et al. 2013), concluding in a monoclonal or few ancestral original clones for these invasive populations.
However, the investigations of Stoeckel et al. (2024) found a high genetic diversity in all populations of Lgh studied despite their predominant clonal reproduction. Interestingly, they found that sexual reproduction is also present, not only in the S-morph by selfing, but also in the L-morph, although limited and preferably by allogamy. They discuss the advantages and drawbacks of the different modes of reproduction observed in Lgh populations, the interactions among them, and the implications that both, the scarcely documented LSI (Gibbs 2014) and selfing, have in the reproductive success and in the maintenance of the high genetic diversity observed in Lgh in western Europe.
The contrasting results with the previous ones (Okada et al. 2009, Armitage et al. 2013) stress the relevance of using appropriate markers and analyses to assess the genetic diversity in autoployploid species, as well as the necessity of knowing the modes of reproduction in the populations studied for an optimal interpretation of the genetic metrics. The approach of the study by Stoeckel et al. (2024) had the challenge of having found suitable markers to deal with a taxon of complex origin such as Lgh, whose genome is compound by a set of autotetraploid chromosomes shared with Lpm and traces of ancient hybridizations of other diploid lineages (Barloy et al. 2024). Using RAD-Seq, Stoeckel et al. (2024) generated an original set of 36 polymorphic SNPs shared between Lgh and Lpm ensuring that these SNPs belong to the tetraploid part of the Lgh genome derived from Lpm. Another interesting contribution of this work is the exhaustive analysis of several genetic descriptors (indexes) and the interpretative guide they provide for each of them in relation to the different modes of reproduction of the study system. Finally, they propose a pair of very useful synthetic indices (i.e., clonality index and selfing index), since they allow to classify populations according to their levels of clonality and selfing.
Stoeckel et al. (2024) conclude the relevance that selfing and LSI populations, and the hybridization between them may have on the expansion and success of invasive plant species, and the necessity to know the modes of reproduction of these populations jointly with their genetic diversity in order to develop appropriate management plans. This study raises new questions such as the modes of reproduction and genetic diversity and structure have other Lgh populations, both invasive and native, and the dynamics of these populations under different future scenarios.
References
Armitage, J. D., Könyves, K., Bailey, J. P., David, J. C., & Culham, A. (2013). A molecular, morphological and cytological investigation of the identity of non-native Ludwigia (Onagraceae) populations in Britain. New Journal of Botany, 3(2), 88–95. https://doi.org/10.1179/2042349713Y.0000000023
Barloy, D., Lemus, L. P.-, Krueger-Hadfield, S. A., Huteau, V., & Coriton, O. (2024). Genomic relationships among diploid and polyploid species of the genus Ludwigia L. section Jussiaea using a combination of cytogenetic, morphological, and crossing investigations. ver. 4 peer-reviewed and recommended by Peer Community in Evolutionary Biology. https://doi.org/10.1101/2023.01.02.522458
Dandelot, S. (2004). Les Ludwigia spp. invasives du Sud de la France: Historique, Biosystématique, Biologie et Ecologie [PhD thesis, Aix-Marseille 3]. https://www.theses.fr/2004AIX30052
Dandelot, S., Verlaque, R., Dutartre, A., & Cazaubon, A. (2005). Ecological, dynamic and taxonomic problems due to Ludwigia (Onagraceae) in France. Hydrobiologia, 551(1), 131–136. https://doi.org/10.1007/s10750-005-4455-0
Gibbs, P. E. (2014). Late-acting self-incompatibility – the pariah breeding system in flowering plants. New Phytologist, 203(3), 717–734. https://doi.org/10.1111/nph.12874
Okada, M., Grewell, B. J., & Jasieniuk, M. (2009). Clonal spread of invasive Ludwigia hexapetala and L. grandiflora in freshwater wetlands of California. Aquatic Botany, 91(3), 123–129. https://doi.org/10.1016/j.aquabot.2009.03.006
Portillo Lemus, L. O., Bozec, M., Harang, M., Coudreuse, J., Haury, J., Stoeckel, S., & Barloy, D. (2021). Self-incompatibility limits sexual reproduction rather than environmental conditions in an invasive water primrose. Plant-Environment Interactions, 2(2), 74–86. https://doi.org/10.1002/pei3.10042
Portillo Lemus, L. O., Harang, M., Bozec, M., Haury, J., Stoeckel, S., & Barloy, D. (2022). Late-acting self-incompatible system, preferential allogamy and delayed selfing in the heteromorphic invasive populations of Ludwigia grandiflora subsp. hexapetala. Peer Community Journal, 2. https://doi.org/10.24072/pcjournal.108
Stoeckel, S., Becheler, R., Portillo-Lemus, L., Harang, M., Besnard, A.-L., Lassalle, G., Causse-Védrines, R., Michon-Coudouel, S., Park D. J., Pope, B. J., Petit, E. J. & Barloy, D. (2024) Reproductive modes in populations of late-acting self-incompatible and self-compatible polyploid Ludwigia grandiflora subsp. hexapetala in western Europe. biorxiv, ver.4 peer-reviewed and recommended by PCI Evol Biol https://doi.org/10.1101/2024.03.21.586104
Transposable Elements are an evolutionary force shaping genomic plasticity in the parthenogenetic root-knot nematode Meloidogyne incognita
DNA transposons drive genome evolution of the root-knot nematode Meloidogyne incognita
Recommended by Ines Alvarez based on reviews by Daniel Vitales and 2 anonymous reviewersDuplications, mutations and recombination may be considered the main sources of genomic variation and evolution. In addition, sexual recombination is essential in purging deleterious mutations and allowing advantageous allelic combinations to occur (Glémin et al. 2019). However, in parthenogenetic asexual organisms, variation cannot be explained by sexual recombination, and other mechanisms must account for it. Although it is known that transposable elements (TE) may influence on genome structure and gene expression patterns, their role as a primary source of genomic variation and rapid adaptability has received less attention. An important role of TE on adaptive genome evolution has been documented for fungal phytopathogens (Faino et al. 2016), suggesting that TE activity might explain the evolutionary dynamics of this type of organisms.
The phytopathogen nematode Meloidogyne incognita is one of the worst agricultural pests in warm climates (Savary et al. 2019). This species, as well as other root-knot nematodes (RKN), shows a wide geographical distribution range infecting diverse groups of plants. Although allopolyploidy may have played an important role on the wide adaptation of this phytopathogen, it may not explain by itself the rapid changes required to overcome plant resistance in a few generations. Paradoxically, M. incognita reproduces asexually via mitotic parthenogenesis (Trudgill and Blok 2001; Castagnone-Sereno and Danchin 2014) and only few single nucleotide variations were identified between different host races isolates (Koutsovoulos et al. 2020). Therefore, this is an interesting model to explore other sources of genomic variation such the TE activity and its role on the success and adaptability of this phytopathogen.
To address these questions, Kozlowski et al. (2020) estimated the TE mobility across 12 geographical isolates that presented phenotypic variations in Meloidogyne incognita, concluding that recent activity of TE in both genic and regulatory regions might have given rise to relevant functional differences between genomes. This was the first estimation of TE activity as a mechanism probably involved in genome plasticity of this root-knot nematode. This study also shed light on evolutionary mechanisms of asexual organisms with an allopolyploid origin. These authors re-annotated the 185 Mb triploid genome of M. incognita for TE content analysis using stringent filters (Kozlowski 2020a), and estimated activity by their distribution using a population genomics approach including isolates from different crops and locations. Canonical TE represented around 4.7% of the M. incognita genome of which mostly correspond to TIR (Terminal Inverted Repeats) and MITEs (Miniature Inverted repeat Transposable Elements) followed by Maverick DNA transposons and LTR (Long Terminal Repeats) retrotransposons. The result that most TE found were represented by DNA transposons is similar to the previous studies with the nematode species model Caenorhabditis elegans (Bessereau 2006; Kozlowski 2020b) and other nematodes as well. Canonical TE annotations were highly similar to their consensus sequences containing transposition machinery when TE are autonomous, whereas no genes involved in transposition were found in non-autonomous ones. These findings suggest recent activity of TE in the M. incognita genome. Other relevant result was the significant variation in TE presence frequencies found in more than 3,500 loci across isolates, following a bimodal distribution within isolates. However, variation in TE frequencies was low to moderate between isolates recapitulating the phylogenetic signal of isolates DNA sequences polymorphisms. A detailed analysis of TE frequencies across isolates allowed identifying polymorphic TE loci, some of which might be neo-insertions mostly of TIRs and MITEs (Kozlowski 2020c). Interestingly, the two thirds of the fixed neo-insertions were located in coding regions or in regulatory regions impacting expression of specific genes in M. incognita. Future research on proteomics is needed to evaluate the functional impact that these insertions have on adaptive evolution in M. incognita. In this line, this pioneer research of Kozlowski et al. (2020) is a first step that is also relevant to remark the role that allopolyploidy and reproduction have had on shaping nematode genomes.
References
[1] Bessereau J-L. 2006. Transposons in C. elegans. WormBook. 10.1895/wormbook.1.70.1
[2] Castagnone-Sereno P, Danchin EGJ. 2014. Parasitic success without sex - the nematode experience. J. Evol. Biol. 27:1323-1333. 10.1111/jeb.12337
[3] Faino L, Seidl MF, Shi-Kunne X, Pauper M, Berg GCM van den, Wittenberg AHJ, Thomma BPHJ. 2016. Transposons passively and actively contribute to evolution of the two-speed genome of a fungal pathogen. Genome Res. 26:1091-1100. 10.1101/gr.204974.116
[4] Glémin S, François CM, Galtier N. 2019. Genome Evolution in Outcrossing vs. Selfing vs. Asexual Species. In: Anisimova M, editor. Evolutionary Genomics: Statistical and Computational Methods. Methods in Molecular Biology. New York, NY: Springer. p. 331-369. 10.1007/978-1-4939-9074-0_11
[5] Koutsovoulos GD, Marques E, Arguel M-J, Duret L, Machado ACZ, Carneiro RMDG, Kozlowski DK, Bailly-Bechet M, Castagnone-Sereno P, Albuquerque EVS, et al. 2020. Population genomics supports clonal reproduction and multiple independent gains and losses of parasitic abilities in the most devastating nematode pest. Evol. Appl. 13:442-457. 10.1111/eva.12881
[6] Kozlowski D. 2020a. Transposable Elements prediction and annotation in the M. incognita genome. Portail Data INRAE. 10.15454/EPTDOS
[7] Kozlowski D. 2020b. Transposable Elements prediction and annotation in the C. elegans genome. Portail Data INRAE. 10.15454/LQCIW0
[8] Kozlowski D. 2020c. TE polymorphisms detection and analysis with PopoolationTE2. Portail Data INRAE. 10.15454/EWJCT8
[9] Kozlowski DK, Hassanaly-Goulamhoussen R, Da Rocha M, Koutsovoulos GD, Bailly-Bechet M, Danchin EG (2020) Transposable Elements are an evolutionary force shaping genomic plasticity in the parthenogenetic root-knot nematode Meloidogyne incognita. bioRxiv, 2020.04.30.069948, ver. 4 peer-reviewed and recommended by PCI Evolutionary Biology. 10.1101/2020.04.30.069948
[10] Savary S, Willocquet L, Pethybridge SJ, Esker P, McRoberts N, Nelson A. 2019. The global burden of pathogens and pests on major food crops. Nat. Ecol. Evol. 3:430-439. 10.1038/s41559-018-0793-y
[11] Trudgill DL, Blok VC. 2001. Apomictic, polyphagous root-knot nematodes: exceptionally successful and damaging biotrophic root pathogens. Annu Rev Phytopathol 39:53-77. 10.1146/annurev.phyto.39.1.53