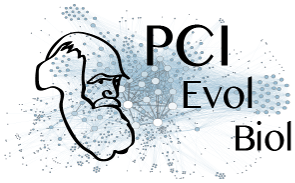
New modelling results help understanding the evolution and maintenance of recombination suppression involving sex chromosomes
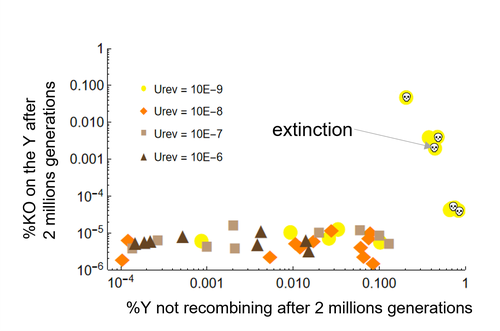
Can mechanistic constraints on recombination reestablishment explain the long-term maintenance of degenerate sex chromosomes?
Abstract
Recommendation: posted 05 February 2024, validated 06 February 2024
Käfer, J. (2024) New modelling results help understanding the evolution and maintenance of recombination suppression involving sex chromosomes. Peer Community in Evolutionary Biology, 100714. 10.24072/pci.evolbiol.100714
Recommendation
Despite advances in genomic research, many views of genome evolution are still based on what we know from a handful of species, such as humans. This also applies to our knowledge of sex chromosomes. We've apparently been too much used to the situation in which a highly degenerate Y chromosome coexists with an almost normal X chromosome to be able to fully grasp all the questions implied by this situation. Lately, many more sex chromosomes have been studied in other organisms, such as in plants, and the view is changing radically: there is a large diversity of situations, ranging from young highly divergent sex chromosomes to old ones that are so similar that they're hard to detect. Undoubtedly inspired by these recent findings, a few theoretical studies have been published around 2 years ago that put an entirely new light on the evolution of sex chromosomes. The differences between these models have however remained somewhat difficult to appreciate by non-specialists.
In particular, the models by Lenormand & Roze (2022) and by Jay et al. (2022) seemed quite similar. Indeed, both rely on the same mechanism for initial recombination suppression: a ``lucky'' inversion, i.e. one with less deleterious mutations than the population average, encompassing the sex-determination locus, is initially selected. However, as it doesn't recombine, it will quickly accumulate deleterious mutations lowering its fitness. And it's at this point the models diverge: according to Lenormand & Roze (2022), nascent dosage compensation not only limits the deleterious effects on fitness by the ongoing degeneration, but it actually opposes recombination restoration as this would lead gene expression away from the optimum that has been reached. On the other hand, in the model by Jay et al. (2022), no additional ingredient is required: they argue that once an inversion had been fixed, reversions that restore recombination are extremely unlikely.
This is what Lenormand & Roze (2024) now call a ``constraint'': in Jay et al.'s model, recombination restoration is impossible for mechanistic reasons. Lenormand & Roze (2024) argue such constraints cannot explain long-term recombination suppression. Instead, a mechanism should evolve to limit the negative fitness effects of recombination arrest, otherwise recombination is either restored, or the population goes extinct due to a dramatic drop in the fitness of the heterogametic sex. These two arguments work together: given the huge fitness cost of the lack of ongoing degeneration of the non-recombining Y, in the absence of compensatory mechanisms, there is a very strong selection for the restoration of recombination, so that even when restoration a priori is orders of magnitude less likely than inversion (leading to recombination suppression), it will eventually happen.
One way the negative fitness effects of recombination suppression can be limited, is the way the authors propose in their own model: dosage compensation evolves through regulatory evolution right at the start of recombination suppression. This changes our classical, simplistic view that dosage compensation evolves in response to degeneration: rather, Lenormand & Roze (2024) argue, that degeneration can only happen when dosage compensation is effective.
The reasoning is convincing and exposes the difference between the models to readers without a firm background in mathematical modelling. Although Lenormand & Roze (2024) target the "constraint theory", it seems likely that other theories for the maintenance of recombination suppression that don't imply the compensation of early degeneration are subject to the same criticism. Indeed, they mention the widely-cited "sexual antagonism" theory, in which mutations with a positive effect in males but a negative in females will select for recombination suppression that will link them to the sex-determining gene on the Y. However, once degeneration starts, the sexually-antagonistic benefits should be huge to overcome the negative effects of degeneration, and it's unlikely they'll be large enough.
A convincing argument by Lenormand & Roze (2024) is that there are many ways recombination could be restored, allowing to circumvent the possible constraints that might be associated with reverting an inversion. First, reversions don't have to be exact to restore recombination. Second, the sex-determining locus can be transposed to another chromosome pair, or an entirely new sex-determining locus might evolve, leading to sex-chromosome turnover which has effectively been observed in several groups.
These modelling studies raise important questions that need to be addressed with both theoretical and empirical work. First, is the regulatory hypothesis proposed by Lenormand & Roze (2022) the only plausible mechanism for the maintenance of long-term recombination suppression? The female- and male-specific trans regulators of gene expression that are required for this model, are they readily available or do they need to evolve first? Both theoretical work and empirical studies of nascent sex chromosomes will help to answer these questions. However, nascent sex chromosomes are difficult to detect and dosage compensation is difficult to reveal.
Second, how many species today actually have "stable" recombination suppression? Maybe many species are in a transient phase, with different populations having different inversions that are either on their way to being fixed or starting to get counterselected. The models have now shown us some possibilities qualitatively but can they actually be quantified to be able to fit the data and to predict whether an observed case of recombination suppression is transient or stable?
The debate will continue, and we need the active contribution of theoretical biologists to help clarify the underlying hypotheses of the proposed mechanisms.
Conflict of interest statement: I did co-author a manuscript with D. Roze in 2023, but do not consider this a conflict of interest. The manuscript is the product of discussions that have taken place in a large consortium mainly in 2019. It furthermore deals with an entirely different topic of evolutionary biology.
References
Jay P, Tezenas E, Véber A, and Giraud T. (2022) Sheltering of deleterious mutations explains the stepwise extension of recombination suppression on sex chromosomes and other supergenes. PLoS Biol.;20:e3001698. https://doi.org/10.1371/journal.pbio.3001698
Lenormand T and Roze D. (2022) Y recombination arrest and degeneration in the absence of sexual dimorphism. Science;375:663-6. https://doi.org/10.1126/science.abj1813
Lenormand T and Roze D. (2024) Can mechanistic constraints on recombination reestablishment explain the long-term maintenance of degenerate sex chromosomes? bioRxiv, ver. 5 peer-reviewed and recommended by Peer Community in Evolutionary Biology. https://doi.org/10.1101/2023.02.17.528909
The recommender in charge of the evaluation of the article and the reviewers declared that they have no conflict of interest (as defined in the code of conduct of PCI) with the authors or with the content of the article. The authors declared that they comply with the PCI rule of having no financial conflicts of interest in relation to the content of the article.
This work is supported by the ANR Genasex and CisTransEvol
Evaluation round #2
DOI or URL of the preprint: https://doi.org/10.1101/2023.02.17.528909
Version of the preprint: 4
Author's Reply, 02 Feb 2024
We corrected the spelling errors in the text, including those mentionned by the editor (except when, after investigating, it was, as far as we could tell, not required).
Decision by Jos Käfer, posted 01 Feb 2024, validated 02 Feb 2024
Dear Thomas Lenormand and Denis Roze,
Thank you for submitting a revised version of the manuscript that addressed most of the comments by the reviewers. I'm suggestion a revision solely to make sure a few errors are corrected before your manuscript is recommended.
- You removed the mention of "heteromorphic sex chromosomes" in the title, I suggest you do the same in the abstract (line 18) for clarity.
- line 41: "has attracted the most attention" -> has attracted most attention
- line 46: "Even if our focus on the long-term" -> Even if *we* focus on the long-term
- line 301: "We use the same simulations than above" -> We use the same simulations *as* above
- line 466: "Investigating whether DC always evolve" -> Investigating whether DC always *evolves*
I suggest you proof-read the text for further errors that might have escaped your and my attention and am looking forward to recommending this manuscript in the next few days on PCI Evol Biol.
Thank you again for your submission,
Jos Käfer
Evaluation round #1
DOI or URL of the preprint: https://doi.org/10.1101/2023.02.17.528909
Version of the preprint: 2
Author's Reply, 18 Jan 2024
Decision by Jos Käfer, posted 22 Dec 2023, validated 23 Dec 2023
Dear Thomas Lenormand and Denis Roze,
Thank you for your submission to PCI Evol Biol. I have received three reviews of your preprint, and all three reviewers agree this is a manuscript of good quality, well-written, and that it is addressing an interesting question. I would thus be delighted to recommend this manuscript on PCI Evol Biol once the comments of the reviewers have been taken into account.
In particular, all three reviewers suggest you include more empirical data on inversions and reversions, and provide some concrete suggestions on how to achieve this.
I also support the suggestion by reviewer 2 to amend the title, as the term "heteromorphic" is ambiguous, and usually applies to differences that can be observed by light microscopy. There indeed appears to be no universal relationship between the size of the non-recombining region and its age (see for example Renner & Müller, Nat. Plants 2021), so old non-recombining regions can be small enough not to be visible under a microscope.
Reviewer 1 suggests you move figure S1 to the main text. While I understand your choice to focus the main text on the critical evaluation of Jay et al.'s model, I do think that showing what additional processes might lead to long-term maintenance of recombination suppression, such as those modeled by yourself, would help clarifying the debate. To this end, I would suggest transforming lines 284-290 into a subsection of its own.
I furthermore suggest you change title of the paragraph "Modelling sex chromosome evolution" into "Methods" and start the "Results" section with the paragraph that follows ("Very low rates of reversion...").
I would like you to consider changing "male fitness" into "fitness of the heterogametic sex" in the paragraph starting at line 249 ("The constraint scenario is more likely to lead to..."). There is a large literature on sex-specific fitness mostly related to fertilization success, offspring production etc., where fitness depends on the type of gametes (small: male; large: female) the individual produces. The fitness effect you're describing occurs in the heterogametic sex, no matter what gametes it produces, and I'm afraid that referring to "male fitness" might be ambiguous for non-specialist readers.
I am confident that the suggestions made by the reviewers will allow you to improve the manuscript even further, and I'm looking forward to reading a revised version.
A few typos not mentioned by the reviewers and suggestions on the text:
- line 217 "a first inversions" -> a first inversion
- line 230 "For example, in the example illustrated on Fig 1B...": redundant formulation. Just "For example, in Fig 1B..." would do.
- line 331 "Fig S4 and S5 illustrates" -> illustrate
Yours sincerely,
Jos Käfer
Reviewed by anonymous reviewer 1, 15 Dec 2023
A general pattern observed across species with sex chromosomes (XX/XY or ZW/ZZ sex determination) is that the sex-specific chromosome (Y or W) and the paired chromosome (X or Z) evolve to suppress recombination along their length, often via structural rearrangements. This is a key transition in the evolution of sex chromosomes, as this shutdown of recombination allows for (1) divergence between the sex chromosome pair and (2) the eventual accumulation of deleterious mutations and loss of gene content on the sex-specific chromosome (‘degradation’). Understanding what causes the initial shutdown of recombination across the sex chromosomes, and what maintains this recombination shutdown, is therefore a key question in the field of sex chromosome evolution.
This paper provides a survey of multiple theories that explain the shutdown of recombination, but focuses on determining whether the recently proposed sheltering theory put forward in Jay et al. 2022 is a viable explanation of recombination. The initial step in the Jay et al. 2022 model involves the fixation of a ‘less-loaded’ or ‘lucky’ inversion: an inversion that happens to capture a genetic region with fewer deleterious mutations than the average haplotype. The dynamics of such inversions on autosomes are well-investigated (Nei et al. 1967). If the inverted haplotype is sufficiently more fit than the corresponding haplotypes, it may fix in the population, which restores recombination in the region captured by the inversion (as homozygotes for the inversion may recombine freely). However, if such an inverted haplotype captures a sex-determining locus (e.g. a dominant male-determining factor, such as SRY in mammals), it remains permanently heterozygous even if it reaches fixation because the male-determining factor is never homozygous (i.e., YY individuals are never produced). The fixation of the inverted haplotype therefore induces a shutdown of recombination in inverted region between the X and Y chromosomes.
Jay et al. 2022 argue that if the 'less-loaded' inversion haplotype also carries recessive deleterious mutations, its presence in a permanently heterozygote form will be beneficial because these deleterious recessive mutations are sheltered from selection in a heterozygote state. As a result, more and more overlapping inversions of this type will accumulate along the Y chromosome, leading to a chromosome-wide shutdown of recombination. However, Jay et al. 2022 assume that the possibility of a reversion of the initial inverted region, which restores recombination between the X and Y, is highly unlikely. This is contrast to a recent model presented in Lenormand and Roze 2022 (the authors of the present paper), which suggested the fixation of such an initial ‘lucky’ inversion is not sufficient to maintain recombination shutdown between the X and Y, because eventually the non-recombining region on the Y will accumulate enough deleterious mutations that a reversion of the inversion will appear and fix.
The focus of the current paper is to determine the rate at which reversions of the initial inverted haplotype must appear to support the Lenormand & Roze model vs. the Jay et al. model. In summary, the authors find that this rate of reversion must be very low relative to the rate of inversions in the population for the initial inversion not to be reverted (approximately 4 orders of magnitude lower than the rate of inversions). The authors argue that additional factors (such as the regulatory divergence on the X and Y proposed in their 2022 paper) are therefore required to maintain the presence of an inversion.
Overall, I found this to be a straightforward paper that clarifies why these two recent and superficially similar models reached such different conclusions about the stability of inversions on the sex chromosomes. While the data on the rate of reversions of inversions is quite limited, this paper will presumably direct more attention to empirical work attempting to measure this parameter. I have some suggestions, given below, for how to strengthen the paper.
--- I feel that the paper would be improved by a more detailed discussion of the molecular biology of inversions (and their potential subsequent reversions). Some of this is provided in lines 353-363, but more background on how & why inversions (and reversions) occur would be very helpful. This is particularly the case in terms of the data on reversions from Drosophila, where there is a proposed mechanism in lines 357-358; this mechanism would increase the probability that a reversion occurs with the same (or similar) breakpoints as the initial inversion, which is the point of contention between the two focal models.
--- A key component of the Jay et al. 2022 is the assumption that deleterious mutations are partially recessive, which allows these mutations to be ‘sheltered’ in their permanently heterozygous form. Here, the dominance of all deleterious mutations is also assumed to be partially recessive (fixed at 0.3). But it would be nice to see a bit more discussion of the implications of this assumption in the paper. For example, if mutations were (on average) even more recessive, would that slow the fitness decline on the inverted region of the Y and allow more time for another inversion to occur (thereby potentially making reversion less likely or slowing the male fitness decline leading to extinction)? Would this also be the case if there was an inverse relationship between h and s as predicted by theories of dominance (e.g. Kacser & Burns 1981, Manna et al. 2011)?
--- Lines 310-313: The authors suggest that the possibility of gene conversions and double-crossovers within the inversions will provide occasional recombination events. Would this then slow the accumulation of deleterious mutations on the Y chromosome? It seems that these occasional recombination events would perhaps stabilize the presence of the inversion on the Y, but simultaneously decrease the rate of degradation of the Y as well (e.g. as in lines 230-240). Further elaboration of the potential impacts of occasional recombination within the inversion on these processes would be helpful.
---Figure S1 – I would suggest moving this to the main text, if there is space; it summarizes the authors data about reversion rate well.
--- Lines 360-363: It would be useful to have citations for this point, as the fact recombination may be suppressed outside the inverted region can certainly make the accumulation of secondary inversions more likely and the possibility of reversion therefore more remote, in line with the Jay et al. 2022 model. E.g. from Drosophila melanogaster, this area of reduced recombination outside the inversion can range between 3-12 mb (Koury 2023)
Kacser, H., & Burns, J. A. (1981). The molecular basis of dominance. Genetics, 97(3-4), 639-666.
Koury, S. A. (2023). Predicting recombination suppression outside chromosomal inversions in Drosophila melanogaster using crossover interference theory. Heredity, 130(4), 196-208.
Manna, F., Martin, G., & Lenormand, T. (2011). Fitness landscapes: an alternative theory for the dominance of mutation. Genetics, 189(3), 923-937.
Nei, M., Kojima, K. I., & Schaffer, H. E. (1967). Frequency changes of new inversions in populations under mutation-selection equilibria. Genetics, 57(4), 741.