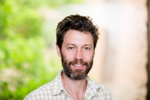
PHILLIPS Ben
- Spatial ecology and evolution laboratory, University of Melbourne, Melbourne, Australia
- Adaptation, Evolutionary Applications, Evolutionary Dynamics, Evolutionary Ecology, Evolutionary Theory, Experimental Evolution, Life History
- recommender
Recommendations: 4
Review: 1
Recommendations: 4
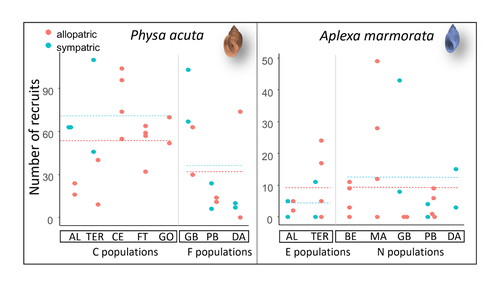
Rapid life-history evolution reinforces competitive asymmetry between invasive and resident species
The evolution of a hobo snail
Recommended by Ben Phillips based on reviews by David Reznick and 2 anonymous reviewersAt the very end of a paper entitled "Copepodology for the ornithologist" Hutchinson (1951) pointed out the possibility of 'fugitive species'. A fugitive species, said Hutchinson, is one that we would typically think of as competitively inferior. Wherever it happens to live it will eventually be overwhelmed by competition from another species. We would expect it to rapidly go extinct but for one reason: it happens to be a much better coloniser than the other species. Now all we need to explain its persistence is a dose of space and a little disturbance: a world in which there are occasional disturbances that cause local extinction of the dominant species. Now, argued Hutchinson, we have a recipe for persistence, albeit of a harried kind. As Hutchinson put it, fugitive species "are forever on the move, always becoming extinct in one locality as they succumb to competition, and always surviving as they reestablish themselves in some other locality."
It is a fascinating idea, not just because it points to an interesting strategy, but also because it enriches our idea of competition: competition for space can be just as important as competition for time.
Hutchinson's idea was independently discovered with the advent of metapopulation theory (Levins 1971; Slatkin 1974) and since then, of course, ecologists have gone looking, and they have unearthed many examples of species that could be said to have a fugitive lifestyle. These fugitive species are out there, but we don't often get to see them evolve.
In their recent paper, Chapuis et al. (2024) make a convincing case that they have seen the evolution of a fugitive species. They catalog the arrival of an invasive freshwater snail on Guadeloupe in the Lesser Antilles, and they wonder what impact this snail's arrival might have on a native freshwater snail. This is a snail invasion, so it has been proceeding at a majestic pace, allowing the researchers to compare populations of the native snail that are completely naive to the invader with those that have been exposed to the invader for either a relatively short period (<20 generations) or longer periods (>20 generations). They undertook an extensive set of competition assays on these snails to find out which species were competitively superior and how the native species' competitive ability has evolved over time.
Against naive populations of the native, the invasive snail turns out to be unequivocally the stronger competitor. (This makes sense; it probably wouldn't have been able to invade if it wasn't.) So what about populations of the native snail that have been exposed for longer, that have had time to adapt? Surprisingly these populations appear to have evolved to become even weaker competitors than they already were.
So why is it that the native species has not simply been driven extinct? Drawing on their previous work on this system, the authors can explain this situation. The native species appears to be the better coloniser of new habitats. Thus, it appears that the arrival of the invasive species has pushed the native species into a different place along the competition-colonisation axis. It has sacrificed competitive ability in favour of becoming a better coloniser; it has become a fugitive species in its own backyard.
This is a really nice empirical study. It is a large lab study, but one that makes careful sampling around a dynamic field situation. Thus, it is a lab study that informs an earlier body of fieldwork and so reveals a fascinating story about what is happening in the field. We are left not only with a particularly compelling example of character displacement towards a colonising phenotype but also with something a little less scientific: the image of a hobo snail, forever on the run, surviving in the spaces in between.
References
Chapuis E, Jarne P, David P (2024) Rapid life-history evolution reinforces competitive asymmetry between invasive and resident species. bioRxiv, 2023.10.25.563987, ver. 2 peer-reviewed and recommended by Peer Community in Evolutionary Biology. https://doi.org/10.1101/2023.10.25.563987
Hutchinson, G.E. (1951) Copepodology for the Ornithologist. Ecology 32: 571–77. https://doi.org/10.2307/1931746
Levins, R., and D. Culver. (1971) Regional Coexistence of Species and Competition between Rare Species. Proceedings of the National Academy of Sciences 68, no. 6: 1246–48. https://doi.org/10.1073/pnas.68.6.1246.
Slatkin, Montgomery. (1974) Competition and Regional Coexistence. Ecology 55, no. 1: 128–34. https://doi.org/10.2307/1934625.
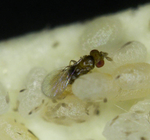
Shifts from pulled to pushed range expansions caused by reduction of landscape connectivity
The push and pull between theory and data in understanding the dynamics of invasion
Recommended by Ben Phillips based on reviews by Laura Naslund and 2 anonymous reviewersExciting times are afoot for those of us interested in the ecology and evolution of invasive populations. Recent years have seen evolutionary process woven firmly into our understanding of invasions (Miller et al. 2020). This integration has inspired a welter of empirical and theoretical work. We have moved from field observations and verbal models to replicate experiments and sophisticated mathematical models. Progress has been rapid, and we have seen science at its best; an intimate discussion between theory and data.
An area currently under very active development is our understanding of pushed invasions. Here a population spreads through space driven, not by dispersal and growth originating at the leading tip of the invasion, but by dispersal and growth originating deeper in the bulk of the population. These pushed invasions may be quite common – they result when per capita growth and dispersal rates are higher in the bulk of the wave than at the leading tip. They result from a range of well-known phenomena, including Allee effects and density-dependent dispersal (Gandhi et al. 2016; Bîrzu et al. 2019). Pushed invasions travel faster than we would expect given growth and dispersal rates on the leading tip, and they lose genetic diversity more slowly than classical pulled invasions (Roques et al. 2012; Haond et al. 2018; Bîrzu et al. 2019).
Well… in theory, anyway. The theory on pushed waves has momentarily streaked ahead of the empirical work, because empirical systems for studying pushed invasions are rare (though see Gandhi et al. 2016; Gandhi, Korolev, and Gore 2019). In this paper, Dahirel and colleagues (2020) make the argument that we may be able to generate pushed invasions in laboratory systems simply by reducing the connectedness of our experimental landscapes. If true, we might have a simple tool for turning many of our established experimental systems into systems for studying pushed dynamics.
It’s a nice idea, and the paper goes to careful lengths to explore the possibility in their lab system (a parasitoid wasp, Trichogramma). They run experiments on replicate wasp populations comparing strongly- v poorly-connected arrays, and estimate the resulting invasion speeds and rate of diversity loss. They also build a simulation model of the system, allowing them to explore in-silico a range of possible processes underlying their results.
As well as developing these parallel systems, Dahirel and colleagues (2020) go to careful lengths to develop statistical analyses that allow inference on key parameters, and they apply these analyses to both the experimental and simulation data. They have been motivated to apply methods that might be used in both laboratory and field settings to help classify invasions.
Ultimately, they found reasonable evidence that their poorly-connected habitat did induce a pushed dynamic. Their poorly connected invasions travelled faster than they should have if they were pulled, they lost diversity more slowly than the highly connected habitat, and replicates with a higher carrying capacity tended to have higher invasion speeds. All in line with expectations of a pushed dynamic. Interestingly, however, their simulation results suggest that they probably got this perfect result for unexpected reasons. The strong hint is that their poorly-connected habitat induced density dependent dispersal in the wasps. Without this effect, their simulations suggest they should have seen diversity decreasing much more rapidly than it did.
There is a nuanced, thoughtful, and carefully argued discussion about all this in the paper, and it is worth reading. There is much of value in this paper. Theirs is not a perfect empirical system in which all the model assumptions are met and in which huge population sizes make stochastic effects negligible. Here is a system one step closer to the messy reality of biology. The struggle to align this system with new theory has been worth the effort. Not only does it give us hope that we might usefully be able to discriminate between classes of invasions using real-world data, but it hints at a rule that Tolstoy might have expressed this way: all pulled invasions are alike, each pushed invasion is pushed in its own way.
References
Bîrzu, G., Matin, S., Hallatschek, O., and Korolev, K. S. (2019). Genetic drift in range expansions is very sensitive to density dependence in dispersal and growth. Ecology Letters, 22(11), 1817-1827. doi: https://doi.org/10.1111/ele.13364
Dahirel, M., Bertin, A., Haond, M., Blin, A., Lombaert, E., Calcagno, V., Fellous, S., Mailleret, L., Malausa, T., and Vercken, E. (2020). Shifts from pulled to pushed range expansions caused by reduction of landscape connectivity. bioRxiv, 2020.05.13.092775, ver. 4 peer-reviewed and recommended by PCI Evolutionary Biology. https://doi.org/10.1101/2020.05.13.092775
Gandhi, S. R., Korolev, K. S., and Gore, J. (2019). Cooperation mitigates diversity loss in a spatially expanding microbial population. Proceedings of the National Academy of Sciences, 116(47), 23582-23587. doi: https://doi.org/10.1073/pnas.1910075116
Gandhi, S. R., Yurtsev, E. A., Korolev, K. S., and Gore, J. (2016). Range expansions transition from pulled to pushed waves as growth becomes more cooperative in an experimental microbial population. Proceedings of the National Academy of Sciences, 113(25), 6922-6927. doi: https://doi.org/10.1073/pnas.1521056113
Haond, M., Morel-Journel, T., Lombaert, E., Vercken, E., Mailleret, L. and Roques, L. (2018). When higher carrying capacities lead to faster propagation (2018), bioRxiv, 307322, ver. 4 peer-reviewed and recommended by Peer Community in Ecology. https://doi.org/10.1101/307322
Miller et al. (2020). Eco‐evolutionary dynamics of range expansion. Ecology, 101(10), e03139. doi: https://doi.org/10.1002/ecy.3139
Roques, L., Garnier, J., Hamel, F., and Klein, E. K. (2012). Allee effect promotes diversity in traveling waves of colonization. Proceedings of the National Academy of Sciences, 109(23), 8828-8833. doi: https://doi.org/10.1073/pnas.1201695109
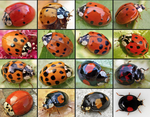
How do invasion syndromes evolve? An experimental evolution approach using the ladybird Harmonia axyridis
Selection on a single trait does not recapitulate the evolution of life-history traits seen during an invasion
Recommended by Inês Fragata and Ben Phillips based on reviews by 2 anonymous reviewersBiological invasions are natural experiments, and often show that evolution can affect dynamics in important ways [1-3]. While we often think of invasions as a conservation problem stemming from anthropogenic introductions [4,5], biological invasions are much more commonplace than this, including phenomena as diverse as natural range shifts, the spread of novel pathogens, and the growth of tumors. A major question across all these settings is which set of traits determine the ability of a population to invade new space [6,7]. Traits such as: increased growth or reproductive rate, dispersal ability and ability to defend from predation often show large evolutionary shifts across invasion history [1,6,8]. Are such multi-trait shifts driven by selection on multiple traits, or a correlated response by multiple traits to selection on one? Resolving this question is important for both theoretical and practical reasons [9,10]. But despite the importance of this issue, it is not easy to perform the necessary manipulative experiments [9].
Foucaud et al. [11] tackled this issue by performing experimental evolution on source populations of the invasive ladybug Harmonia axyridis. The authors tested if selection on a single trait could generate correlated responses in other life history traits. Specifically, they used experimental evolution to impose divergent selection on female mass, and reproductive timing. After ten generations, they found that selection for weight did not affect almost any other life history trait. However, nine generations of selection for faster reproduction led to correlated phenotypic changes in developmental, reproduction and survival rate of populations, although not always in the direction we might have expected. Despite this correlated response, none of their selected lines were able to fully recapitulate the trait shifts seen in natural invasions of this species. This implies that selection during natural invasions is operating on multiple traits; a finding in agreement with our growing understanding of how selection acts during introduction and invasion [12,13].
Populations undergoing a colonization process may also be subject to a multitude of different selective pressures [14,15]. The authors expanded their work in this direction by testing whether food availability alters the observed correlations between life history traits. The pervasiveness of genotype by environment interactions observed also points to a role for multiple selective pressures in shaping the suite of life-history shifts observed in wild ladybug populations. The work from Foucaud and colleagues [11] adds to a small but growing list of important studies that use experimental evolution to investigate how life-history traits evolve, and how they evolve during invasions in particular.
References
[1] Sakai, A.K., Allendorf, F.W., Holt, J.S. et al. (2001). The population biology of invasive species. Annual review of ecology and systematics, 32(1), 305-332. doi: 10.1146/annurev.ecolsys.32.081501.114037
[2] Hairston Jr, N. G., Ellner, S. P., Geber, M. A., Yoshida, T. and Fox, J. A. (2005). Rapid evolution and the convergence of ecological and evolutionary time. Ecology letters, 8(10), 1114-1127. doi: 10.1111/j.1461-0248.2005.00812.x
[3] Chuang, A. and Peterson, C. R. (2016). Expanding population edges: theories, traits, and trade‐offs. Global change biology, 22(2), 494-512. doi: 10.1111/gcb.13107
[4] Whitney, K. D. and Gabler, C. A. (2008). Rapid evolution in introduced species,‘invasive traits’ and recipient communities: challenges for predicting invasive potential. Diversity and Distributions, 14(4), 569-580. doi: 10.1111/j.1472-4642.2008.00473.x
[5] Catullo, R. A., Llewelyn, J., Phillips, B. L. and Moritz, C. C. (2019). The Potential for Rapid Evolution under Anthropogenic Climate Change. Current Biology, 29(19), R996-R1007. doi: 10.1016/j.cub.2019.08.028
[6] Suarez, A. V. and Tsutsui, N. D. (2008). The evolutionary consequences of biological invasions. Molecular Ecology, 17(1), 351-360. doi: 10.1111/j.1365-294X.2007.03456.x
[7] Deforet, M., Carmona-Fontaine, C., Korolev, K. S. and Xavier, J. B. (2019). Evolution at the edge of expanding populations. The American Naturalist, 194(3), 291-305. doi: 10.1086/704594
[8] Phillips, B. L., Brown, G. P., and Shine, R. (2010). Life‐history evolution in range‐shifting populations. Ecology, 91(6), 1617-1627. doi: 10.1890/09-0910.1
[9] Colautti, R. I. and Lau, J. A. (2015). Contemporary evolution during invasion: evidence for differentiation, natural selection, and local adaptation. Molecular ecology, 24(9), 1999-2017. doi: 10.1111/mec.13162
[10] Szűcs, M., Melbourne, B. A., Tuff, T., Weiss‐Lehman, C. and Hufbauer, R. A. (2017). Genetic and demographic founder effects have long‐term fitness consequences for colonising populations. Ecology Letters, 20(4), 436-444. doi: 10.1111/ele.12743
[11] Foucaud, J., Hufbauer, R. A., Ravigné, V., Olazcuaga, L., Loiseau, A., Ausset, A., Wang, S., Zang, L.-S., Lemenager, N., Tayeh, A., Weyna, A., Gneux, P., Bonnet, E., Dreuilhe, V., Poutout, B., Estoup, A. and Facon, B. (2020). How do invasion syndromes evolve? An experimental evolution approach using the ladybird Harmonia axyridis. bioRxiv, 849968 ver. 4 peer-reviewed and recommended by PCI Evolutionary Biology. doi: 10.1101/849968
[12] Simons, A. M. (2003). Invasive aliens and sampling bias. Ecology Letters, 6(4), 278-280. doi: 10.1046/j.1461-0248.2003.00430.x
[13] Phillips, B. L. and Perkins, T. A. (2019). Spatial sorting as the spatial analogue of natural selection. Theoretical Ecology, 12(2), 155-163. doi: 10.1007/s12080-019-0412-9
[14] Lavergne, S. and Molofsky, J. (2007). Increased genetic variation and evolutionary potential drive the success of an invasive grass. Proceedings of the National Academy of Sciences, 104(10), 3883-3888. doi: 10.1073/pnas.0607324104
[15] Moran, E. V. and Alexander, J. M. (2014). Evolutionary responses to global change: lessons from invasive species. Ecology Letters, 17(5), 637-649. doi: 10.1111/ele.12262
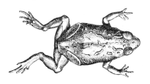
Geographic variation in adult and embryonic desiccation tolerance in a terrestrial-breeding frog
Tough as old boots: amphibians from drier habitats are more resistant to desiccation, but less flexible at exploiting wet conditions
Recommended by Ben Phillips based on reviews by Juan Diego Gaitan-Espitia, Jennifer Nicole Lohr and 1 anonymous reviewerSpecies everywhere are facing rapid climatic change, and we are increasingly asking whether populations will adapt, shift, or perish [1]. There is a growing realisation that, despite limited within-population genetic variation, many species exhibit substantial geographic variation in climate-relevant traits. This geographic variation might play an important role in facilitating adaptation to climate change [2,3].
Much of our understanding of geographic variation in climate-relevant traits comes from model organisms [e.g. 4]. But as our concern grows, we make larger efforts to understand geographic variation in non-model organisms also. If we understand what adaptive geographic variation exists within a species, we can make management decisions around targeted gene flow [5]. And as empirical examples accumulate, we can look for generalities that can inform management of unstudied species [e.g. 6,7]. Rudin-Bitterli’s paper [8] is an excellent contribution in this direction.
Rudin-Bitterli and her co-authors [8] sampled six frog populations distributed across a strong rainfall gradient. They then assayed these frogs and their offspring for a battery of fitness-relevant traits. The results clearly show patterns consistent with local adaptation to water availability, but they also reveal trade-offs. In their study, frogs from the driest source populations were resilient to the hydric environment: it didn’t really affect them very much whether they were raised in wet or dry environments. By contrast, frogs from wet source areas did better in wet environments, and they tended to do better in these wet environments than did animals from the dry-adapted populations. Thus, it appears that the resilience of the dry-adapted populations comes at a cost: frogs from these populations cannot ramp up performance in response to ideal (wet) conditions.
These data have been carefully and painstakingly collected, and they are important. They reveal not only important geographic variation in response to hydric stress (in a vertebrate), but they also adumbrate a more general trade-off: that the jack of all trades might be master of none. Specialist-generalist trade-offs are often argued (and regularly observed) to exist [e.g. 9,10], and here we see them arise in climate-relevant traits also. Thus, Rudin-Bitterli’s paper is an important piece of the empirical puzzle, and one that points to generalities important for both theory and management.
References
[1] Hoffmann, A. A., and Sgrò, C. M. (2011). Climate change and evolutionary adaptation. Nature, 470(7335), 479–485. doi: 10.1038/nature09670
[2] Aitken, S. N., and Whitlock, M. C. (2013). Assisted Gene Flow to Facilitate Local Adaptation to Climate Change. Annual Review of Ecology, Evolution, and Systematics, 44(1), 367–388. doi: 10.1146/annurev-ecolsys-110512-135747
[3] Kelly, E., and Phillips, B. L. (2016). Targeted gene flow for conservation. Conservation Biology, 30(2), 259–267. doi: 10.1111/cobi.12623
[4] Sgrò, C. M., Overgaard, J., Kristensen, T. N., Mitchell, K. A., Cockerell, F. E., and Hoffmann, A. A. (2010). A comprehensive assessment of geographic variation in heat tolerance and hardening capacity in populations of Drosophila melanogaster from eastern Australia. Journal of Evolutionary Biology, 23(11), 2484–2493. doi: 10.1111/j.1420-9101.2010.02110.x
[5] Macdonald, S. L., Llewelyn, J., and Phillips, B. L. (2018). Using connectivity to identify climatic drivers of local adaptation. Ecology Letters, 21(2), 207–216. doi: 10.1111/ele.12883
[6] Hoffmann, A. A., Chown, S. L., and Clusella‐Trullas, S. (2012). Upper thermal limits in terrestrial ectotherms: how constrained are they? Functional Ecology, 27(4), 934–949. doi: 10.1111/j.1365-2435.2012.02036.x
[7] Araújo, M. B., Ferri‐Yáñez, F., Bozinovic, F., Marquet, P. A., Valladares, F., and Chown, S. L. (2013). Heat freezes niche evolution. Ecology Letters, 16(9), 1206–1219. doi: 10.1111/ele.12155
[8] Rudin-Bitterli, T. S., Evans, J. P., and Mitchell, N. J. (2019). Geographic variation in adult and embryonic desiccation tolerance in a terrestrial-breeding frog. BioRxiv, 314351, ver. 3 peer-reviewed and recommended by Peer Community in Evolutionary Biology. doi: 10.1101/314351
[9] Kassen, R. (2002). The experimental evolution of specialists, generalists, and the maintenance of diversity. Journal of Evolutionary Biology, 15(2), 173–190. doi: 10.1046/j.1420-9101.2002.00377.x
[10] Angilletta, M. J. J. (2009). Thermal Adaptation: A theoretical and empirical synthesis. Oxford University Press, Oxford.
Review: 1
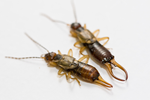
Thermal regimes, but not mean temperatures, drive patterns of rapid climate adaptation at a continent-scale: evidence from the introduced European earwig across North America
Temperature variance, rather than mean, drives adaptation to local climate
Recommended by Fabien Aubret based on reviews by Ben Phillips and Eric GangloffClimate change is impacting eco-systems worldwide and driving many populations to move, adapt or go extinct. It is increasingly appreciated, for example, that species may adjust their phenology in response to climate change, although empirical data is scarce. In this preprint [1], Tourneur and Meunier report an impressive sampling effort in which life-history traits were measured across introduced populations of earwig in North America. The authors examine whether variation in life-history across populations is correlated with aspects of the thermal climate experienced by each population: mean temperature and seasonality of temperature. They find some fascinating correlations between life-history and thermal climate; correlations with the seasonality of temperature, but not with mean temperature. This study provides relatively uncommon data, in the sense that where most of the literature looking at adaptation in animals in response to climate change has focused on physiological traits [2, 3], this study examines changes in life-history traits with time scales relevant to impending climate change, and provides a reasonable argument that this is adaptation, not just constraint.
References
[1] Tourneur, J.-C. and Meunier, J. (2019). Thermal regimes, but not mean temperatures, drive patterns of rapid climate adaptation at a continent-scale: evidence from the introduced European earwig across North America. BioRxiv, 550319, ver. 4 peer-reviewed and recommended by PCI Evolutionary Biology. doi: 10.1101/550319
[2] Kellermann, V., Overgaard, J., Hoffmann, A. A., Fløjgaard, C., Svenning, J. C., & Loeschcke, V. (2012). Upper thermal limits of Drosophila are linked to species distributions and strongly constrained phylogenetically. Proceedings of the National Academy of Sciences, 109(40), 16228-16233. doi: 10.1073/pnas.1207553109
[3] Hoffmann, A. A., & Sgro, C. M. (2011). Climate change and evolutionary adaptation. Nature, 470(7335), 479. doi: 10.1038/nature09670